1. Chemical context
The structural chemistry of organotin(IV) compounds with multidentate Schiff base ligands has been of interest since the observation of the diversity in their supramolecular association patterns (Teoh et al., 1997
; Dey et al., 1999
). Typically, these multidentate ligands bind to the tin atom through the phenolic-O, imine-N, oxime-O or even oxime-N atoms. In view of this, the coordination of these multidentate ligands to (organo)tin may lead to more thermodynamically stable organotin complexes, in contrast to those with monodentate ligands (Vallet et al., 2003
; Contreras et al., 2009
), a feature which could potentially be useful in catalytic studies (Yearwood et al., 2002
). In consideration of this and as part of on-going work with multidentate ligands of organotin compounds (Lee et al., 2004
), an attempt to synthesize an adduct of the potentially tetradentate Schiff base N,N-1,1,2,2-dinitrilevinylenebis(5-bromosalicylaldiminato) with di(p-fluorobenzyl)tin(IV) dichloride was made.
The complex was obtained as an orange powder and was successfully characterized using various spectroscopic methods including 1H NMR spectroscopy. Upon interaction with DMSO-d6, in the context of NMR studies, colourless crystals were obtained after several weeks standing. The formation of the new title compound, (I)
, is likely due to degradation of the complex while stored in the NMR tube. In the present contribution, the crystal and molecular structures of (I)
are described as well as a detailed analysis of the intermolecular association through a Hirshfeld surface analysis.
2. Structural commentary
The molecular structure of (I)
, Fig. 1
, has the SnIV atom situated on a crystallographic centre of inversion. The SnIV atom is coordinated by monodentate ligands, i.e. chloride, sulfoxide-O and methylene-C atoms. From symmetry, each donor is trans to a like atom resulting in an all-trans-C2Cl2O2 donor set about the SnIV atom. The donor set defines a distorted octahedral geometry owing, in part, to the disparate Sn—donor atom bond lengths, Table 1
. The angles about the SnIV atom differ relatively little from the ideal octahedral angles with the maximum deviation of ca 6° noted for the C1—Sn—O1 angle, Table 1
.
Sn—C1 | 2.1628 (16) | Sn—Cl1 | 2.5599 (4) | Sn—O1 | 2.2332 (11) | | | | | | | C1—Sn—O1 | 95.99 (5) | C1—Sn—Cl1i | 89.95 (5) | C1—Sn—Cl1 | 90.05 (5) | O1—Sn—Cl1 | 90.44 (3) | C1—Sn—O1i | 84.01 (5) | O1—Sn—Cl1i | 89.56 (3) | Symmetry code: (i) -x+1, -y+1, -z+1. | |
| Figure 1 The molecular structure of (I) , showing the atom-labelling scheme and displacement ellipsoids at the 70% probability level. The SnIV atom lies on a centre of inversion; unlabelled atoms are related by the symmetry operation 1 − x, 1 − y, 1 − z. |
3. Supramolecular features
The molecular packing in (I)
comprises C—H⋯F, C—H⋯Cl and C—H⋯π interactions which combine to generate a three-dimensional network, Table 2
. The chloride atom participates in phenyl-C6—H⋯Cl1 and methyl-C8—H⋯Cl1 interactions. As each chloride atom is involved in two C—H⋯Cl interactions and there are two chloride atoms per molecule, the C—H⋯Cl interactions extend laterally to give rise to a supramolecular layer in the bc plane, Fig. 2
a. Layers are connected along the a axis by phenyl-C3—H⋯F1 and methyl-C9—H⋯π(phenyl) interactions to consolidate the molecular packing, Fig. 2
b.
D—H⋯A | D—H | H⋯A | D⋯A | D—H⋯A | C3—H3⋯F1ii | 0.95 | 2.49 | 3.333 (2) | 147 | C6—H6⋯Cl1iii | 0.95 | 2.77 | 3.6129 (18) | 148 | C8—H8B⋯Cl1iv | 0.98 | 2.76 | 3.6379 (17) | 150 | C9—H9C⋯Cg1v | 0.98 | 2.67 | 3.3887 (19) | 130 | Symmetry codes: (ii) ; (iii) ; (iv) ; (v) x+1, y, z. | |
| Figure 2 The molecular packing in (I) : (a) supramolecular layer in the bc plane sustained by C—H⋯Cl interactions and (b) a view of the unit-cell contents in projection down the c axis. The C—H⋯Cl, C—H⋯F and C—H⋯π interactions are shown as orange, blue and purple dashed lines, respectively. |
4. Hirshfeld surface analysis
The Hirshfeld surface analysis on the structure of (I)
provides more insight into the molecular packing and was performed as described recently (Wardell et al., 2016
). It is evident from the bright-red spots appearing near the chloride and fluoride atoms on the Hirshfeld surface mapped over dnorm in Fig. 3
that these atoms play a significant role in the molecular packing. Thus, the bright-red spots near phenyl-H6, methyl-H8B and a pair near Cl1 in Fig. 3
indicate the presence of bifurcated C—H⋯Cl interactions formed by each of the chloride atoms. Similarly, the pair of red spots near phenyl-H3 and F1 atoms are associated with the donor and acceptor of C—H⋯F interactions, respectively. The donors and acceptors of C—H⋯Cl and C—H⋯F interactions are also represented with blue (positive potential) and red regions (negative potential), respectively, on the Hirshfeld surface mapped over the electrostatic potential in Fig. 4
. In addition to above, the Cl1 and F1 atoms also participate in short interatomic contacts with methyl-H atoms, Table 3
. The presence of faint-red spots near the phenyl-C4 and methyl-C9 atoms in Fig. 3
indicate their participation in a short interatomic C⋯C contact, Table 3
, which compliments the methyl-C—H⋯π(phenyl) contact described above. The presence of the C—H⋯π interaction is also evident from the view of Hirshfeld surface mapped over the electrostatic potential around participating atoms, Fig. 4
; the donors and acceptors of these interactions are viewed as the convex surface around atoms of the methyl-C9 groups and the concave surface above the (C2–C7) phenyl ring, respectively. The immediate environments about a reference molecule within dnorm- and shape-index-mapped Hirshfeld surfaces highlighting the various C—H⋯Cl, C—H⋯F and C—H⋯π interactions are illustrated in Fig. 5
a–c, respectively.
Contact | distance | symmetry operation | C4⋯C9 | 3.371 (2) | −1 + x, y, z | F1⋯H8A | 2.66 | 1 − x, −y, 1 − z | Cl1⋯H9B | 2.91 | 1 − x, + y, − z | Note: (a) the SnIV atom is located on a centre of inversion. | |
| Figure 3 A view of the Hirshfeld surface for (I) mapped over dnorm over the range −0.049 to 1.356 au. |
| Figure 4 A view of the Hirshfeld surface for (I) mapped over the electrostatic potential in the range ±0.095 au. |
| Figure 5 Views of the Hirshfeld surfaces about a reference molecule mapped over (a) shape-index, (b) dnorm and (c) shape-index, highlighting (a) C—H⋯F and short interatomic F⋯H/H⋯F contacts as black and red dashed lines, respectively, (b) C—H⋯Cl and short interatomic Cl⋯H/H⋯Cl contacts as black and red dashed lines, respectively, and (c) C—H⋯π and short interatomic C⋯C contacts as white and red dashed lines, respectively. |
The overall two-dimensional fingerprint plot and those delineated into H⋯H, Cl⋯H/H⋯Cl, F⋯H/H⋯F, C⋯H/H⋯C and O⋯H/H⋯O contacts (McKinnon et al., 2007
) are illustrated in Fig. 6
a–f, respectively, and their relative contributions to the Hirshfeld surfaces are summarized in Table 4
. It is clear from the fingerprint plot delineated into H⋯H contacts, Fig. 6
b, that although these contacts have the greatest contribution, i.e. 45.7%, to the Hirshfeld surface, the dispersion forces acting between them keep these atoms at the distances greater than the sum of their van der Waals radii, hence they do not contribute significantly to the molecular packing. The comparatively greater contribution of F⋯H/H⋯F contacts to the Hirshfeld surface cf. Cl⋯H/H⋯Cl contacts, Table 4
, is due to the relative positions of the chloride and fluoride atoms in the molecule, the fluoride atoms being at the extremities and the chloride atoms near the tin(IV) atom. However, the Cl⋯H/H⋯Cl contacts have a greater influence on the molecular packing as viewed from the delineated fingerprint plot in Fig. 6
c. The forceps-like distribution of points in the plot with tips at de + di ∼2.8 Å result from the bifurcated C—H⋯Cl interactions, and points at positions less than the sum of their van der Waals radii are ascribed to the short interatomic Cl⋯H/H⋯Cl contacts, the green appearance due to high density of interactions. Similarly, a pair of short spikes at de + di ∼2.5 Å in the fingerprint plot delineated into F⋯H/H⋯F contacts, Fig. 6
d, are indicative of intermolecular C—H⋯F interactions with the short interatomic F⋯H/H⋯F contacts merged within the fingerprint plot. It is important to note from the fingerprint plot delineated into C⋯H/H⋯C contacts, Fig. 6
e, that even though their interatomic distances are equal to or greater than the sum of their van der Waals radii, i.e. 2.9 Å, the 12.8% contribution from these to the Hirshfeld surfaces are indicative of the presence of C—H⋯π interactions in the structure. This is also justified from the presence of short interatomic C⋯C contacts, Fig. 5
c and Table 3
. The 4.1% contribution from O⋯H/H⋯O contributions to Hirshfeld surfaces, Fig. 6
f, and the small contributions from the other contacts listed in Table 2
have a negligible effect on the packing.
Contact | percentage contribution | H⋯H | 45.7 | Cl⋯H/H⋯Cl | 15.1 | F⋯H/H⋯F | 19.8 | C⋯H/H⋯C | 12.8 | O⋯H/H⋯O | 4.1 | S⋯H/H⋯S | 1.7 | Cl⋯F/F⋯Cl | 0.6 | C⋯Cl/Cl⋯C | 0.1 | F⋯F | 0.1 | | |
| Figure 6 Fingerprint plots for (I) : (a) overall and those delineated into (b) H⋯H, (c) Cl⋯H/H⋯Cl, (d) F⋯H/H⋯F, (e) C⋯H/H⋯C and (f) O⋯H/H⋯O contacts. |
6. Synthesis and crystallization
All chemicals and solvents were used as purchased without purification. Di(p-fluorobenzyl)tin dichloride was prepared in accordance with the literature method (Sisido et al., 1961
). All reactions were carried out under ambient conditions. The melting point was determined using an Electrothermal digital melting point apparatus and was uncorrected. The IR spectrum was obtained on a Perkin Elmer Spectrum 400 FT Mid-IR/Far-IR spectrophotometer in the range 4000 to 400 cm−1. The 1H NMR spectrum was recorded at room temperature in CDCl3 solution on a Jeol ECA 400 MHz FT–NMR spectrometer.
N,N′-1,1,2,2-Dinitrilevinylenebis(5-bromosalicylaldiminato) (1.0 mmol, 0.401 g; prepared by the condensation reaction between diaminomaleonitrile and 5-bromosalicylaldehyde in a 2:1 molar ratio in ethanol) and triethylamine (1.0 mmol, 0.14 ml) in ethyl acetate (25 ml) was added to di(p-fluorobenzyl)tin dichloride (1.0 mmol, 0.183 g) in ethyl acetate (10 ml). The resulting mixture was stirred and refluxed for 4 h. The filtrate was evaporated until a dark-orange precipitate was obtained. The precipitate was dissolved in DMSO-d6 solution in a NMR tube for 1H NMR spectroscopic characterization. After the analysis, the tube was set aside for a month and colourless crystals of (I)
suitable for X-ray crystallographic studies were obtained from the slow evaporation. Yield: 0.060 g, 11%; m.p: 399 K. IR (cm−1): 1595(m) ν(C=C), 1504(s) ν(S=O), 1161(m), 578(w), 508(m) ν(Sn—O). 1H NMR (in CDCl3): 6.90–7.11, 7.35–7.40 (m, 8H, aromatic-H), 3.11 (s, 6H, –CH3), 2.17 (m, 4H, –CH2).
Crystal data, data collection and structure refinement details are summarized in Table 6
. Carbon-bound H-atoms were placed in calculated positions (C—H = 0.95–0.99 Å) and were included in the refinement in the riding model approximation, with Uiso(H) set to 1.2–1.5Ueq(C).
Crystal data | Chemical formula | [Sn(C7H6F)2Cl2(C2H6OS)2] | Mr | 564.08 | Crystal system, space group | Monoclinic, P21/c | Temperature (K) | 100 | a, b, c (Å) | 8.2363 (1), 12.7020 (2), 11.4038 (1) | β (°) | 110.391 (2) | V (Å3) | 1118.28 (3) | Z | 2 | Radiation type | Cu Kα | μ (mm−1) | 13.28 | Crystal size (mm) | 0.24 × 0.12 × 0.10 | | Data collection | Diffractometer | Agilent SuperNova, Dual, Cu at zero, AtlasS2 | Absorption correction | Multi-scan (CrysAlis PRO; Rigaku Oxford Diffraction, 2015 ) | Tmin, Tmax | 0.636, 1.000 | No. of measured, independent and observed [I > 2σ(I)] reflections | 7792, 2292, 2228 | Rint | 0.020 | (sin θ/λ)max (Å−1) | 0.631 | | Refinement | R[F2 > 2σ(F2)], wR(F2), S | 0.018, 0.046, 1.08 | No. of reflections | 2292 | No. of parameters | 126 | H-atom treatment | H-atom parameters constrained | Δρmax, Δρmin (e Å−3) | 0.35, −0.76 | Computer programs: CrysAlis PRO (Rigaku Oxford Diffraction, 2015 ), SHELXS (Sheldrick, 2008 ), SHELXL2014 (Sheldrick, 2015 ), ORTEP-3 for Windows (Farrugia, 2012 ), DIAMOND (Brandenburg, 2006 ) and publCIF (Westrip, 2010 ). | |
Supporting information
Data collection: CrysAlis PRO (Rigaku Oxford Diffraction, 2015); cell refinement: CrysAlis PRO (Rigaku Oxford Diffraction, 2015); data reduction: CrysAlis PRO (Rigaku Oxford Diffraction, 2015); program(s) used to solve structure: SHELXS (Sheldrick, 2008); program(s) used to refine structure: SHELXL2014 (Sheldrick, 2015); molecular graphics: ORTEP-3 for Windows (Farrugia, 2012) and DIAMOND (Brandenburg, 2006); software used to prepare material for publication: publCIF (Westrip, 2010).
trans-Dichloridobis(dimethyl sulfoxide-
κO)bis(4-fluorobenzyl-
κC1)tin(IV)
top Crystal data top [Sn(C7H6F)2Cl2(C2H6OS)2] | F(000) = 564 |
Mr = 564.08 | Dx = 1.675 Mg m−3 |
Monoclinic, P21/c | Cu Kα radiation, λ = 1.54184 Å |
a = 8.2363 (1) Å | Cell parameters from 6127 reflections |
b = 12.7020 (2) Å | θ = 5.4–76.3° |
c = 11.4038 (1) Å | µ = 13.28 mm−1 |
β = 110.391 (2)° | T = 100 K |
V = 1118.28 (3) Å3 | Prism, colourless |
Z = 2 | 0.24 × 0.12 × 0.10 mm |
Data collection top Agilent SuperNova, Dual, Cu at zero, AtlasS2 diffractometer | 2292 independent reflections |
Radiation source: micro-focus sealed X-ray tube, SuperNova (Cu) X-ray Source | 2228 reflections with I > 2σ(I) |
Mirror monochromator | Rint = 0.020 |
ω scans | θmax = 76.5°, θmin = 5.4° |
Absorption correction: multi-scan (CrysAlis PRO; Rigaku Oxford Diffraction, 2015) | h = −10→9 |
Tmin = 0.636, Tmax = 1.000 | k = −15→15 |
7792 measured reflections | l = −14→14 |
Refinement top Refinement on F2 | 0 restraints |
Least-squares matrix: full | Hydrogen site location: inferred from neighbouring sites |
R[F2 > 2σ(F2)] = 0.018 | H-atom parameters constrained |
wR(F2) = 0.046 | w = 1/[σ2(Fo2) + (0.0233P)2 + 0.7527P] where P = (Fo2 + 2Fc2)/3 |
S = 1.08 | (Δ/σ)max < 0.001 |
2292 reflections | Δρmax = 0.35 e Å−3 |
126 parameters | Δρmin = −0.76 e Å−3 |
Special details top Geometry. All esds (except the esd in the dihedral angle between two l.s. planes) are estimated using the full covariance matrix. The cell esds are taken into account individually in the estimation of esds in distances, angles and torsion angles; correlations between esds in cell parameters are only used when they are defined by crystal symmetry. An approximate (isotropic) treatment of cell esds is used for estimating esds involving l.s. planes. |
Fractional atomic coordinates and isotropic or equivalent isotropic displacement parameters (Å2) top | x | y | z | Uiso*/Ueq | |
Sn | 0.5000 | 0.5000 | 0.5000 | 0.00673 (6) | |
Cl1 | 0.44665 (6) | 0.48762 (3) | 0.26545 (4) | 0.01508 (10) | |
S1 | 0.55778 (5) | 0.24628 (3) | 0.43015 (3) | 0.00881 (9) | |
F1 | 0.06456 (15) | 0.03219 (9) | 0.34347 (11) | 0.0248 (2) | |
O1 | 0.60349 (15) | 0.33583 (9) | 0.52752 (10) | 0.0106 (2) | |
C1 | 0.2332 (2) | 0.45714 (13) | 0.46551 (16) | 0.0130 (3) | |
H1A | 0.2054 | 0.4746 | 0.5410 | 0.016* | |
H1B | 0.1583 | 0.5013 | 0.3962 | 0.016* | |
C2 | 0.1878 (2) | 0.34437 (13) | 0.43339 (15) | 0.0098 (3) | |
C3 | 0.1093 (2) | 0.31260 (13) | 0.30871 (15) | 0.0111 (3) | |
H3 | 0.0838 | 0.3637 | 0.2440 | 0.013* | |
C4 | 0.0679 (2) | 0.20772 (14) | 0.27783 (15) | 0.0134 (3) | |
H4 | 0.0147 | 0.1868 | 0.1930 | 0.016* | |
C5 | 0.1058 (2) | 0.13487 (13) | 0.37280 (16) | 0.0140 (3) | |
C6 | 0.1822 (2) | 0.16192 (14) | 0.49747 (16) | 0.0144 (3) | |
H6 | 0.2062 | 0.1101 | 0.5613 | 0.017* | |
C7 | 0.2229 (2) | 0.26727 (14) | 0.52668 (15) | 0.0121 (3) | |
H7 | 0.2758 | 0.2873 | 0.6118 | 0.014* | |
C8 | 0.6371 (2) | 0.13286 (13) | 0.52466 (15) | 0.0154 (3) | |
H8A | 0.7576 | 0.1447 | 0.5786 | 0.023* | |
H8B | 0.6312 | 0.0718 | 0.4708 | 0.023* | |
H8C | 0.5662 | 0.1197 | 0.5766 | 0.023* | |
C9 | 0.7181 (2) | 0.25482 (14) | 0.35875 (16) | 0.0159 (3) | |
H9A | 0.7035 | 0.3209 | 0.3118 | 0.024* | |
H9B | 0.7055 | 0.1952 | 0.3017 | 0.024* | |
H9C | 0.8335 | 0.2530 | 0.4235 | 0.024* | |
Atomic displacement parameters (Å2) top | U11 | U22 | U33 | U12 | U13 | U23 |
Sn | 0.00785 (9) | 0.00543 (9) | 0.00565 (8) | 0.00005 (4) | 0.00078 (6) | −0.00080 (4) |
Cl1 | 0.0208 (2) | 0.0153 (2) | 0.00719 (18) | 0.00143 (14) | 0.00249 (16) | 0.00013 (13) |
S1 | 0.00860 (18) | 0.00802 (18) | 0.00877 (16) | 0.00079 (13) | 0.00171 (14) | −0.00157 (13) |
F1 | 0.0250 (6) | 0.0089 (5) | 0.0328 (6) | −0.0026 (4) | 0.0006 (5) | −0.0014 (5) |
O1 | 0.0135 (6) | 0.0067 (5) | 0.0095 (5) | 0.0016 (4) | 0.0012 (4) | −0.0021 (4) |
C1 | 0.0082 (7) | 0.0131 (9) | 0.0172 (8) | −0.0007 (6) | 0.0039 (6) | −0.0030 (6) |
C2 | 0.0059 (7) | 0.0112 (8) | 0.0122 (7) | 0.0000 (6) | 0.0032 (6) | −0.0012 (6) |
C3 | 0.0087 (7) | 0.0129 (8) | 0.0105 (7) | 0.0005 (6) | 0.0018 (6) | 0.0023 (6) |
C4 | 0.0115 (8) | 0.0152 (9) | 0.0117 (7) | −0.0004 (6) | 0.0020 (6) | −0.0028 (6) |
C5 | 0.0110 (8) | 0.0078 (8) | 0.0211 (8) | −0.0014 (6) | 0.0030 (6) | −0.0018 (6) |
C6 | 0.0117 (8) | 0.0151 (8) | 0.0152 (8) | 0.0015 (6) | 0.0032 (6) | 0.0065 (6) |
C7 | 0.0089 (8) | 0.0176 (8) | 0.0090 (7) | −0.0006 (6) | 0.0022 (6) | 0.0000 (6) |
C8 | 0.0228 (9) | 0.0080 (8) | 0.0141 (8) | 0.0027 (6) | 0.0049 (7) | 0.0002 (6) |
C9 | 0.0166 (9) | 0.0175 (9) | 0.0167 (8) | 0.0005 (6) | 0.0097 (7) | −0.0019 (6) |
Geometric parameters (Å, º) top Sn—C1 | 2.1628 (16) | C3—C4 | 1.390 (2) |
Sn—C1i | 2.1628 (16) | C3—H3 | 0.9500 |
Sn—O1 | 2.2332 (11) | C4—C5 | 1.375 (2) |
Sn—O1i | 2.2332 (11) | C4—H4 | 0.9500 |
Sn—Cl1i | 2.5599 (4) | C5—C6 | 1.383 (2) |
Sn—Cl1 | 2.5599 (4) | C6—C7 | 1.392 (2) |
S1—O1 | 1.5417 (11) | C6—H6 | 0.9500 |
S1—C9 | 1.7796 (17) | C7—H7 | 0.9500 |
S1—C8 | 1.7815 (17) | C8—H8A | 0.9800 |
F1—C5 | 1.360 (2) | C8—H8B | 0.9800 |
C1—C2 | 1.494 (2) | C8—H8C | 0.9800 |
C1—H1A | 0.9900 | C9—H9A | 0.9800 |
C1—H1B | 0.9900 | C9—H9B | 0.9800 |
C2—C7 | 1.400 (2) | C9—H9C | 0.9800 |
C2—C3 | 1.401 (2) | | |
| | | |
C1—Sn—C1i | 180.0 | C4—C3—C2 | 121.27 (15) |
C1—Sn—O1 | 95.99 (5) | C4—C3—H3 | 119.4 |
C1—Sn—Cl1 | 90.05 (5) | C2—C3—H3 | 119.4 |
C1—Sn—Cl1i | 89.95 (5) | C5—C4—C3 | 118.50 (15) |
C1—Sn—O1i | 84.01 (5) | C5—C4—H4 | 120.8 |
C1—Sn—Cl1i | 89.95 (5) | C3—C4—H4 | 120.8 |
O1—Sn—Cl1 | 90.44 (3) | F1—C5—C4 | 118.88 (15) |
O1—Sn—Cl1i | 89.56 (3) | F1—C5—C6 | 118.46 (15) |
C1i—Sn—O1 | 84.01 (5) | C4—C5—C6 | 122.66 (16) |
C1i—Sn—O1i | 95.99 (5) | C5—C6—C7 | 118.07 (15) |
O1—Sn—O1i | 180.0 | C5—C6—H6 | 121.0 |
C1i—Sn—Cl1i | 90.05 (5) | C7—C6—H6 | 121.0 |
O1i—Sn—Cl1i | 90.44 (3) | C6—C7—C2 | 121.47 (15) |
O1i—Sn—Cl1 | 89.56 (3) | C6—C7—H7 | 119.3 |
Cl1i—Sn—Cl1 | 180.000 (18) | C2—C7—H7 | 119.3 |
O1—S1—C9 | 104.51 (8) | S1—C8—H8A | 109.5 |
O1—S1—C8 | 102.41 (7) | S1—C8—H8B | 109.5 |
C9—S1—C8 | 98.73 (8) | H8A—C8—H8B | 109.5 |
S1—O1—Sn | 127.03 (6) | S1—C8—H8C | 109.5 |
C2—C1—Sn | 115.97 (11) | H8A—C8—H8C | 109.5 |
C2—C1—H1A | 108.3 | H8B—C8—H8C | 109.5 |
Sn—C1—H1A | 108.3 | S1—C9—H9A | 109.5 |
C2—C1—H1B | 108.3 | S1—C9—H9B | 109.5 |
Sn—C1—H1B | 108.3 | H9A—C9—H9B | 109.5 |
H1A—C1—H1B | 107.4 | S1—C9—H9C | 109.5 |
C7—C2—C3 | 118.03 (15) | H9A—C9—H9C | 109.5 |
C7—C2—C1 | 121.12 (14) | H9B—C9—H9C | 109.5 |
C3—C2—C1 | 120.85 (15) | | |
| | | |
C9—S1—O1—Sn | 92.64 (10) | C3—C4—C5—F1 | 179.52 (15) |
C8—S1—O1—Sn | −164.80 (9) | C3—C4—C5—C6 | 0.4 (3) |
Sn—C1—C2—C7 | 81.29 (17) | F1—C5—C6—C7 | −179.60 (15) |
Sn—C1—C2—C3 | −98.42 (16) | C4—C5—C6—C7 | −0.5 (3) |
C7—C2—C3—C4 | −0.3 (2) | C5—C6—C7—C2 | 0.1 (3) |
C1—C2—C3—C4 | 179.42 (15) | C3—C2—C7—C6 | 0.2 (2) |
C2—C3—C4—C5 | 0.0 (2) | C1—C2—C7—C6 | −179.50 (15) |
Symmetry code: (i) −x+1, −y+1, −z+1. |
Hydrogen-bond geometry (Å, º) topCg1 is the centroid of the C2–C7 ring. |
D—H···A | D—H | H···A | D···A | D—H···A |
C3—H3···F1ii | 0.95 | 2.49 | 3.333 (2) | 147 |
C6—H6···Cl1iii | 0.95 | 2.77 | 3.6129 (18) | 148 |
C8—H8B···Cl1iv | 0.98 | 2.76 | 3.6379 (17) | 150 |
C9—H9C···Cg1v | 0.98 | 2.67 | 3.3887 (19) | 130 |
Symmetry codes: (ii) −x, y+1/2, −z+1/2; (iii) x, −y−1/2, z−1/2; (iv) −x+1, y−1/2, −z+1/2; (v) x+1, y, z. |
Summary of short interatomic contacts (Å) in (I) topContact | distance | symmetry operation |
C4···C9 | 3.371 (2) | -1 + x, y, z |
F1···H8A | 2.66 | 1 - x, -y, 1 - z |
Cl1···H9B | 2.91 | 1 - x, 1/2 + y, 1/2 - z |
Note: (a) the SnIV atom is located on a centre of inversion. |
Percentage contribution of interatomic contacts to the Hirshfeld surface for (I) topContact | percentage contribution |
H···H | 45.7 |
Cl···H/H···Cl | 15.1 |
F···H/H···F | 19.8 |
C···H/H···C | 12.8 |
O···H/H···O | 4.1 |
S···H/H···S | 1.7 |
Cl···F/F···Cl | 0.6 |
C···Cl/Cl···C | 0.1 |
F···F | 0.1 |
Selected geometric parameters (Å, °) for molecules of the general formula R2SnX2(DMSO)2 topCompound | X—Sn—X | O—Sn—O | C—Sn—C | Reference |
Me2SnBr2(DMSO)2 | 180 | 180 | 180 | Aslanov et al. (1978) |
Me2SnCl2(DMSO)2 | 95.2 (3) | 83.7 (5) | 172.7 (3) | Aslanov et al. (1978) |
Ph2SnCl2(DMSO)2 | 97.43 (3) | 79.34 (9) | 172.17 (14) | Sadiq-ur-Rehman et al. (2007) |
(4-FC6H4CH2)2SnCl2(DMSO)2 | 180 | 180 | 180 | This work |
Funding information
Funding for this research was provided by: Sunway University (award No. INT-RRO-2017–096); University of Malaya (award Nos. RP017A-2014AFR, PG239–2016\#65532;A); Ministry of Higher Education, Malaysia (MOHE) Fundamental Research Grant Scheme (award No. FP033–2014B).
References
Aslanov, L. A., Ionov, V. M., Attiya, V. M., Permin, A. B. & Petrosyan, V. S. (1978). Zh. Strukt. Khim. 19, 109–115. CAS Google Scholar
Brandenburg, K. (2006). DIAMOND. Crystal Impact GbR, Bonn, Germany. Google Scholar
Contreras, R., Flores-Parra, A., Mijangos, E., Téllez, F., López-Sandoval, H. & Barba-Behrens, N. (2009). Coord. Chem. Rev. 253, 1979–1999. Web of Science CrossRef CAS Google Scholar
Dey, D. K., Dasa, M. K. & Nöth, H. (1999). Z. Naturforsch. Teil B, 54, 145–154. CAS Google Scholar
Farrugia, L. J. (2012). J. Appl. Cryst. 45, 849–854. Web of Science CrossRef CAS IUCr Journals Google Scholar
Groom, C. R., Bruno, I. J., Lightfoot, M. P. & Ward, S. C. (2016). Acta Cryst. B72, 171–179. Web of Science CSD CrossRef IUCr Journals Google Scholar
Isaacs, N. W. & Kennard, C. H. L. (1970). J. Chem. Soc. A, pp. 1257–1261. CSD CrossRef Web of Science Google Scholar
Lee, S. M., Lo, K. M. & Ng, S. W. (2004). Acta Cryst. E60, m1614–m1616. Web of Science CSD CrossRef IUCr Journals Google Scholar
McKinnon, J. J., Jayatilaka, D. & Spackman, M. A. (2007). Chem. Commun. pp. 3814–3816. Web of Science CrossRef Google Scholar
Rigaku Oxford Diffraction (2015). CrysAlis PRO. Agilent Technologies Inc., Santa Clara, CA, USA. Google Scholar
Sadiq-ur-Rehman, Saeed, S., Ali, S., Shahzadi, S. & Helliwell, M. (2007). Acta Cryst. E63, m1788. Web of Science CSD CrossRef IUCr Journals Google Scholar
Sheldrick, G. M. (2008). Acta Cryst. A64, 112–122. Web of Science CrossRef CAS IUCr Journals Google Scholar
Sheldrick, G. M. (2015). Acta Cryst. C71, 3–8. Web of Science CrossRef IUCr Journals Google Scholar
Sisido, K., Takeda, Y. & Kinugawa, Z. (1961). J. Am. Chem. Soc. 83, 538–541. CrossRef Web of Science Google Scholar
Teoh, S.-G., Yeap, G.-Y., Loh, C.-C., Foong, L.-W., Teo, S.-B. & Fun, H.-K. (1997). Polyhedron, 16, 2213–2221. CSD CrossRef CAS Web of Science Google Scholar
Vallet, V., Wahlgren, U. & Grenthe, I. (2003). J. Am. Chem. Soc. 125, 14941–14950. Web of Science CrossRef PubMed CAS Google Scholar
Wardell, J. L., Jotani, M. M. & Tiekink, E. R. T. (2016). Acta Cryst. E72, 1618–1627. Web of Science CSD CrossRef IUCr Journals Google Scholar
Westrip, S. P. (2010). J. Appl. Cryst. 43, 920–925. Web of Science CrossRef CAS IUCr Journals Google Scholar
Yearwood, B., Parkin, S. & Atwood, D. A. (2002). Inorg. Chim. Acta, 333, 124–131. Web of Science CSD CrossRef CAS Google Scholar
This is an open-access article distributed under the terms of the Creative Commons Attribution (CC-BY) Licence, which permits unrestricted use, distribution, and reproduction in any medium, provided the original authors and source are cited.
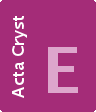 | CRYSTALLOGRAPHIC COMMUNICATIONS |
ISSN: 2056-9890
Open

access