1. Chemical context
In the realm of coordination polymers/metal–organic framework structures, bridging bipyridyl ligands have proven most effective in connecting metal centres. This is equally true in the construction of coordination polymers of cadmium(II) dithiocarbamates, Cd(S2CNR2)2, R = alkyl. Thus, one-dimensional polymers have been found in the crystals of [Cd(S2CNR2)2(NN)]n in cases where R = Et and NN = 1,2-bis(4-pyridyl)ethylene (Chai et al., 2003
), R = Et and NN = 1,2-bis(4-pyridyl)ethane (Avila et al., 2006
) and R = Benz, NN = 4,4′-bipyridyl (Fan et al., 2007
). In an extension of these studies, hydrogen-bonding functionality, in the form of hydroxyethyl groups was included in at least one of the R groups of Cd(S2CNR2)2. It was of some surprise that coordination polymers based on Cd←N dative bonds were not formed as the putative bridging NN ligand was terminally bound. The first example of this phenomenon was noted in a compound closely related to the title compound, i.e. Cd[S2CN(n-Pr)CH2CH2OH)]2(4-pyridinealdazine)2 (Broker & Tiekink, 2011
), for which both potentially bidentate ligands are monodentate. The non-coordinating pyridyl-N atoms participate in hydroxyl-O—H⋯N(pyridyl) hydrogen-bonds. In another interesting example, regardless of the stoichiometry of the reaction between Cd[S2CN(i-Pr)CH2CH2OH]2 and 1,2-bis(4-pyridyl)ethylene, i.e. 1:2, 1:1 and 2:1, only the binuclear compound {Cd[S2CN(i-Pr)CH2CH2OH)]2}2[1,2-bis(4-pyridyl)ethylene]3, featuring one bridging and two terminally bound 1,2-bis(4-pyridyl)ethylene ligands, could be isolated (Jotani et al., 2016
). Finally, in an unprecedented result, the original binuclear {Cd[S2CN(i-Pr)CH2CH2OH]2}2 aggregate was retained in the structure of [{Cd[S2CN(i-Pr)CH2CH2OH]2}2(3-pyridinealdazine)]2 with two terminally bound 3-pyridinealdazine ligands (Arman et al., 2016
). This is unusual as there are no precedents of adduct formation by the zinc-triad dithiocarbamates that resulted in the retention of the original binuclear core (Tiekink, 2003
).
By contrast to the chemistry described above for cadmium dithiocarbamates, no polymeric structures have been observed for zinc analogues with potentially bridging bipyridyl molecules. Instead, only binuclear compounds of the general formula [Zn(S2CNRR′)2]2(NN), i.e. R = CH2CH2OH and R′ = Me, Et or CH2CH2OH for NN = 4,4′-bipyridyl (Benson et al., 2007
), R = R′ = CH2CH2OH and NN = pyrazine (Jotani et al., 2017
), and R = CH2CH2OH and R′ = Me for NN = (3-pyridyl)CH2N(H)C(=Y)C(=Y)N(H)CH2(3-pyridyl) where Y = O (Poplaukhin & Tiekink, 2010
) and Y = S (Poplaukhin et al., 2012
). There are also several all-alkyl species adopting the binuclear motif with a notable example being the product of the reaction of [Zn(S2CNR2)2]2 with an excess of 1,2-bis(4-pyridyl)ethylene in which the binuclear species co-crystallized with an uncoordinated molecule of 1,2-bis(4-pyridyl)ethylene (Lai & Tiekink, 2003
). This difference in behaviour, i.e. polymer formation for cadmium but not for zinc dithiocarbamates, is explained in terms of the larger size of cadmium versus zinc, which enables cadmium to increase its coordination number. In continuation of our studies in this area, the title compound, Zn[S2CN(Me)CH2CH2OH)]2(4-pyridinealdazine), (I)
, was isolated and shown to feature a terminally bound 4-pyridinealdazine ligand. Herein, its crystal and molecular structures are described as is an analysis of the calculated Hirshfeld surface.
3. Supramolecular features
Both conventional and non-conventional hydrogen-bonding interactions feature in the crystal of (I)
, Table 2
. Hydroxyl-O—H⋯O(hydroxyl) hydrogen-bonds between centrosymmetrically related molecules lead to 28-membered {⋯HOC2NCSZnSCNC2O}2 synthons. On either side of this aggregate are hydroxyl-O—H⋯N(pyridyl) hydrogen bonds leading to centrosymmetric 40-membered {⋯HOC2NCSZnNC4N2C4N}2 synthons. The result is a supramolecular double-chain with the appearance of a ladder that extends along [1
0], Fig. 2
a. Within the chains there are notable methylene-C—H⋯π(chelate ring) interactions, Table 2
, which are garnering greater attention in the chemical crystallographic community (Tiekink, 2017
). While the hydroxyl-O2 atom participates in acceptor O—H⋯O and donor O—H⋯N hydrogen-bonds, the O1 atom only forms a O—H⋯O hydrogen-bond. This being stated, this atom accepts a close pyridyl-C—H interaction so that each chain is associated with four other chains. As seen from Fig. 2
b, the surrounding chains are inclined by approximately 90° and have orientations orthogonal to the reference chain. In this manner, a three-dimensional architecture is constructed as illustrated in Fig. 2
c.
D—H⋯A | D—H | H⋯A | D⋯A | D—H⋯A | O1—H1O⋯O2i | 0.85 (5) | 1.92 (5) | 2.721 (5) | 158 (5) | O2—H2O⋯N6ii | 0.84 (4) | 1.95 (4) | 2.769 (5) | 163 (5) | C20—H20⋯O1iii | 0.95 | 2.32 | 3.233 (6) | 162 | C6—H6B⋯Cg1i | 0.99 | 2.59 | 3.540 (4) | 162 | Symmetry codes: (i) -x, -y+1, -z+1; (ii) -x+1, -y-1, -z+1; (iii) . | |
| Figure 2 Molecular packing for (I) : (a) the supramolecular double chain sustained by O—H⋯O and O—H⋯N hydrogen-bonding, shown as orange and blue, dashed lines, respectively, (b) a view of the immediate environment of one chain down the direction of propagation highlighting the role of C—H⋯O interactions (purple dashed lines) in sustaining the three-dimensional architecture and (c) a view of the unit-cell contents in projection down the b axis. |
4. Hirshfeld surface analysis
Additional insight into the intermolecular interactions influential in the crystal of (I)
was obtained from an analysis of the Hirshfeld surfaces which were calculated in accord with a recent publication on related zinc dithiocarbamate compounds (Jotani et al., 2017
). On the Hirshfeld surface mapped over dnorm, Fig. 3
, the donors and acceptors of the O—H⋯O and O—H⋯N hydrogen-bonds are viewed as bright-red spots near hydroxyl-H1O, H2O, hydroxyl-O2 and pyridyl-N6 atoms, located largely at the extremes of the molecule. The presence of bright-red spots near the H1O and H2O atoms in Fig. 3
are also indicative of short inter-atomic H⋯H and C⋯H/H⋯C contacts, see Table 3
. The diminutive-red spots near the methyl-C14, sulfur-S4, pyridyl-H20 and hydroxyl-O1 atoms characterize the influence of short inter-atomic C⋯S/S⋯C contacts, Table 3
, and intermolecular pyridine-C20—H20⋯O1 interactions. The donors and acceptors of the above intermolecular interactions are also represented with blue and red regions on the Hirshfeld surface mapped over electrostatic potential shown in Fig. 4
. The immediate environments about a reference molecule within dnorm-mapped Hirshfeld surface highlighting intermolecular O—H⋯O, O—H⋯N and C—H⋯O, short inter-atomic C⋯S/S⋯C contacts, π—π stacking interactions and C—H⋯π(chelate) interactions are illustrated in Fig. 5
a–c, respectively.
Contact | Distance | Symmetry operation | H1O⋯H2O | 2.21 (7) | −x, 1 − y, 1 − z | H4B⋯H13 | 2.30 | −x, + y, − z | Zn⋯C6 | 3.835 (4) | −x, 1 − y, 1 − z | Zn⋯H6B | 3.00 | −x, 1 − y, 1 − z | C1⋯H6B | 2.88 | −x, 1 − y, 1 − z | S1⋯H6B | 2.92 | −x, 1 − y, 1 − z | S1⋯H15 | 2.98 | x, 1 + y, z | S2⋯H7B | 2.89 | −x, −y, 1 − z | S4⋯C14 | 3.217 (4) | x, 1 + y, z | C2⋯H4A | 2.88 | −x, − + y, − z | C5⋯H18 | 2.77 | 1 − x, 1 − y, 1 − z | C18⋯H2O | 2.89 (5) | 1 − x, 1 − y, 1 − z | C19⋯H2O | 2.85 (4) | 1 − x, 1 − y, 1 − z | N5⋯H8A | 2.73 | 1 − x, −y, 1 − z | | |
| Figure 3 Two views of the Hirshfeld surface for (I) mapped over dnorm in the range −0.400 to 1.552 au. |
| Figure 4 Two views of the Hirshfeld surface for (I) mapped over the electrostatic potential in the range ±0.151 au. The red and blue regions represent negative and positive electrostatic potentials, respectively. |
| Figure 5 Views of Hirshfeld surface mapped over dnorm about a reference molecule showing (a) intermolecular O—H⋯O, O—H⋯N and C—H⋯O interactions as black dashed lines, (b) short inter-atomic S⋯C/C⋯S contacts and π—π stacking interactions as black and red lines, respectively (H atoms are omitted) and (c) C—H⋯π(chelate) interactions through short inter-atomic contacts involving the methylene-H6B atom with the Zn, S1 and C1 atoms of the chelate ring as black dashed lines. |
The overall two dimensional fingerprint plot, Fig. 6
a, and those delineated into H⋯H, C⋯H/H⋯C, N⋯H/H⋯N, S⋯H/H⋯S, O⋯H/H⋯O, C⋯C, C⋯S/S⋯C and Zn⋯H/H⋯Zn contacts (McKinnon et al., 2007
) are illustrated in Fig. 6
b–i, respectively; the relative contributions from different inter-atomic contacts to the Hirshfeld surfaces are summarized in Table 4
. The pair of adjacent short spikes at de + di ∼ 2.2 Å flanked by the broad spikes with tips at de + di ∼ 2.3 Å in the fingerprint plot delineated into H⋯H contacts are due to short inter-atomic H⋯H contacts, Fig. 6
b. The forceps-like tips at de + di ∼ 2.8 Å in the fingerprint plot delineated into C⋯H/H⋯C contacts, Fig. 6
c, are due to the presence of some short inter-atomic contacts involving these atoms, Table 3
. The effect of the intermolecular C—H⋯π(chelate) interactions is also reflected by the short inter-atomic contacts formed by the methylene-C6 with the Zn atom, and methylene-H6B with the Zn, S1 and C1 atoms of the chelate ring, Fig. 6
c, 6e, 6i, and Table 2
. The two pairs of adjacent long spikes on the fingerprint plots delineated into N⋯H/H⋯N and O⋯H/H⋯O contacts, Fig. 6
d and 6f, with the pair of tips at de + di ∼ 2.0 Å and de + di ∼ 1.9 Å, respectively, indicate the presence of conventional O—H⋯O and O—H⋯N hydrogen-bonds in the structure. The points corresponding to short inter-atomic N⋯H/H⋯N contacts, Table 3
, are merged within the plot in Fig. 6
d. The pattern of aligned green points superimposed on the forceps-like distribution of blue points in the S⋯H/H⋯S delineated fingerprint plot in Fig. 6
e characterize the presence of short inter-atomic S⋯H/H⋯S contacts, Table 3
, and C—H⋯π (chelate) interactions, Fig. 5
c. The C—H⋯O interactions appear as the distribution of points in the short parabolic form attached to each of the spikes on the outer side of fingerprint plot delineated into O⋯H/H⋯O contacts, Fig. 6
f, with (de + di)min ∼ 2.3 Å. The parabolic distribution of points in the (de = di) ∼ 1.8–2.0 Å range in the fingerprint plot delineated into C⋯C contacts, Fig. 6
g, indicate the existence of weak π–π stacking interactions between the pyridyl-(N3,C9–C13) and (N6, C15–C20)i rings [Cg⋯Cgi = 3.901 (3) Å; symmetry code: (i) = x, 1 + y, z]. This observation is also viewed as the flat region around these rings in the Hirshfeld surfaces mapped over curvedness in Fig. 7
. Both the C⋯S/S⋯C and Zn⋯H/H⋯Zn contacts make small but discernible contributions of 1.2 and 0.6% to the Hirshfeld surface, respectively, which are manifested as the pair of the short spikes in the centre of Fig. 6
h, with their tips at de + di ∼ 3.2 Å, and wings in Fig. 6
i. The low contribution from other contacts summarized in Table 4
have no significant influence on the molecular packing owing to their long separations.
Contact | Percentage contribution | H⋯H | 44.6 | S⋯H/H⋯S | 15.4 | C⋯H/H⋯C | 13.1 | N⋯H/H⋯N | 10.2 | O⋯H/H⋯O | 6.7 | C⋯C | 2.8 | S⋯N/N⋯S | 2.8 | S⋯S | 1.5 | C⋯S/S⋯C | 1.2 | C⋯N/N⋯C | 1.0 | Zn⋯H/H⋯Zn | 0.6 | Zn⋯S/S⋯Zn | 0.1 | | |
| Figure 6 The full two-dimensional fingerprint plot for (I) and fingerprint plots delineated into (b) H⋯H, (c) C⋯H/H⋯C, (d) N⋯H/H⋯N, (e) S⋯H/H⋯S, (f) O⋯H/H⋯O, (g) C⋯C, (h) C⋯S/S⋯C and (i) Zn⋯H/H⋯Zn contacts. |
| Figure 7 Two views of Hirshfeld surface mapped over curvedness showing flat regions over pyridyl-(N3,C9–C13) and (N6, C15–C20) rings with labels 1 and 2, respectively. |
5. Database survey
A search of the Cambridge Structural Database (Version 5.38, May 2017 update; Groom et al., 2016
) showed there were over 145 examples of metal complexes/main-group element compounds containing the 4-pyridinealdazine molecule. Bridging modes were observed in both cadmium(II) (Lai & Tiekink, 2006
) and nickel(II) (e.g. Berdugo & Tiekink, 2009
) dithiophosphate [−S2P(OR)2] derivatives, indicating bridging modes are possible in the presence of 1,1-dithiolate co-ligands. There were six examples of structures where 4-pyridinealdazine was present in the crystal but was non-coordinating, and two where the ligand was terminally bound as in (I)
, i.e. the cadmium analogue of (I)
and in a structure particularly worth highlighting as both a terminally bound ligand as well as a non-coordinating molecule of 4-pyridinealdazine are present, namely [Zn(OH2)2[O(H)Me]2(4-pyridinealdazine)2](ClO4)2·4-pyridinealdazine, 1.72MeOH, 1.28H2O (Shoshnik et al., 2005
). In summary, the 4-pyridinealdazine molecule is usually found to be bridging, a conclusion vindicated by this mode of coordination being observed in about 95% of structures having 4-pyridinealdazine. While one might be tempted to ascribe the unusual behaviour of 4-pyridinealdazine in (I)
and the cadmium(II) analogue to the influence of hydrogen-bonding associated with the dithiocarbamate ligand, it is salutatory to recall that the sole example of a monodentate bipyridyl ligand is found in the structure of Zn[S2CN(n-Pr)2]2(4,4′-bipyridyl) (Klevtsova et al., 2001
), where there is no possibility of conventional hydrogen-bonding interactions; the binuclear species, {Zn[S2CN(n-Pr)2]2}2(4,4′-bipyridyl), was characterized in the same study.
Crystal data, data collection and structure refinement details are summarized in Table 5
. The carbon-bound H atoms were placed in calculated positions (C—H = 0.95–0.99 Å) and were included in the refinement in the riding-model approximation, with Uiso(H) set to 1.2–1.5Ueq(C). The O-bound H atoms were located in a difference-Fourier map but were refined with distance restraint of O—H = 0.84±0.01 Å, and with Uiso(H) set to 1.5Ueq(O).
Crystal data | Chemical formula | [Zn(C4H8NOS2)2C12H10N4)] | Mr | 576.08 | Crystal system, space group | Monoclinic, P21/c | Temperature (K) | 153 | a, b, c (Å) | 11.499 (4), 8.5710 (19), 25.945 (7) | β (°) | 95.515 (8) | V (Å3) | 2545.3 (13) | Z | 4 | Radiation type | Mo Kα | μ (mm−1) | 1.32 | Crystal size (mm) | 0.40 × 0.18 × 0.15 | | Data collection | Diffractometer | Rigaku AFC12K/SATURN724 | Absorption correction | Multi-scan (ABSCOR; Higashi, 1995 ) | Tmin, Tmax | 0.575, 1 | No. of measured, independent and observed [I > 2σ(I)] reflections | 25373, 4485, 4180 | Rint | 0.044 | (sin θ/λ)max (Å−1) | 0.595 | | Refinement | R[F2 > 2σ(F2)], wR(F2), S | 0.050, 0.132, 1.13 | No. of reflections | 4485 | No. of parameters | 306 | No. of restraints | 2 | H-atom treatment | H atoms treated by a mixture of independent and constrained refinement | Δρmax, Δρmin (e Å−3) | 0.72, −0.44 | Computer programs: CrystalClear (Molecular Structure Corporation & Rigaku, 2005 ), SHELXS (Sheldrick, 2008 ), SHELXL2014/7 (Sheldrick, 2015 ), ORTEP-3 for Windows (Farrugia, 2012 ) and DIAMOND (Brandenburg, 2006 ), publCIF (Westrip, 2010 ). | |
Supporting information
Data collection: CrystalClear (Molecular Structure Corporation & Rigaku, 2005); cell refinement: CrystalClear (Molecular Structure Corporation & Rigaku, 2005); data reduction: CrystalClear (Molecular Structure Corporation & Rigaku, 2005); program(s) used to solve structure: SHELXS (Sheldrick, 2008); program(s) used to refine structure: SHELXL2014/7 (Sheldrick, 2015); molecular graphics: ORTEP-3 for Windows (Farrugia, 2012) and DIAMOND (Brandenburg, 2006); software used to prepare material for publication: publCIF (Westrip, 2010).
Bis[
N-2-hydroxyethyl,
N-methyldithiocarbamato-
κ2S,
S)'-4-{[(pyridin-4-ylmethylidene)hydrazinylidene}methyl]pyridine-
κN1)zinc(II)
top Crystal data top [Zn(C4H8NOS2)2C12H10N4)] | F(000) = 1192 |
Mr = 576.08 | Dx = 1.503 Mg m−3 |
Monoclinic, P21/c | Mo Kα radiation, λ = 0.71069 Å |
a = 11.499 (4) Å | Cell parameters from 1535 reflections |
b = 8.5710 (19) Å | θ = 3.1–30.3° |
c = 25.945 (7) Å | µ = 1.32 mm−1 |
β = 95.515 (8)° | T = 153 K |
V = 2545.3 (13) Å3 | Prism, yellow |
Z = 4 | 0.40 × 0.18 × 0.15 mm |
Data collection top Rigaku AFC12K/SATURN724 diffractometer | 4485 independent reflections |
Radiation source: fine-focus sealed tube | 4180 reflections with I > 2σ(I) |
Graphite monochromator | Rint = 0.044 |
ω scans | θmax = 25.0°, θmin = 2.3° |
Absorption correction: multi-scan (ABSCOR; Higashi, 1995) | h = −13→13 |
Tmin = 0.575, Tmax = 1 | k = −10→8 |
25373 measured reflections | l = −30→30 |
Refinement top Refinement on F2 | 2 restraints |
Least-squares matrix: full | Hydrogen site location: mixed |
R[F2 > 2σ(F2)] = 0.050 | H atoms treated by a mixture of independent and constrained refinement |
wR(F2) = 0.132 | w = 1/[σ2(Fo2) + (0.0663P)2 + 3.198P] where P = (Fo2 + 2Fc2)/3 |
S = 1.13 | (Δ/σ)max = 0.001 |
4485 reflections | Δρmax = 0.72 e Å−3 |
306 parameters | Δρmin = −0.44 e Å−3 |
Special details top Geometry. All esds (except the esd in the dihedral angle between two l.s. planes) are estimated using the full covariance matrix. The cell esds are taken into account individually in the estimation of esds in distances, angles and torsion angles; correlations between esds in cell parameters are only used when they are defined by crystal symmetry. An approximate (isotropic) treatment of cell esds is used for estimating esds involving l.s. planes. |
Fractional atomic coordinates and isotropic or equivalent isotropic displacement parameters (Å2) top | x | y | z | Uiso*/Ueq | |
Zn | 0.13806 (4) | 0.15577 (5) | 0.42882 (2) | 0.03058 (16) | |
S1 | 0.13958 (8) | 0.27822 (11) | 0.34505 (4) | 0.0337 (2) | |
S2 | −0.04566 (8) | 0.07162 (11) | 0.37564 (4) | 0.0327 (2) | |
S3 | 0.04838 (8) | 0.20445 (11) | 0.50658 (4) | 0.0332 (2) | |
S4 | 0.26964 (8) | 0.34274 (11) | 0.48156 (4) | 0.0349 (2) | |
O1 | −0.2678 (3) | 0.3986 (4) | 0.25882 (13) | 0.0546 (8) | |
H1O | −0.225 (4) | 0.462 (5) | 0.277 (2) | 0.082* | |
O2 | 0.1675 (3) | 0.4188 (3) | 0.66339 (11) | 0.0447 (7) | |
H2O | 0.230 (3) | 0.373 (6) | 0.658 (2) | 0.067* | |
N1 | −0.0546 (3) | 0.2180 (4) | 0.28469 (12) | 0.0346 (7) | |
N2 | 0.1419 (3) | 0.4612 (3) | 0.55174 (11) | 0.0305 (7) | |
N3 | 0.2411 (3) | −0.0416 (4) | 0.42704 (12) | 0.0325 (7) | |
N4 | 0.4206 (3) | −0.5525 (4) | 0.38299 (13) | 0.0377 (8) | |
N5 | 0.4931 (3) | −0.6843 (4) | 0.38951 (13) | 0.0363 (7) | |
N6 | 0.6562 (3) | −1.2152 (4) | 0.35773 (14) | 0.0437 (8) | |
C1 | 0.0057 (3) | 0.1899 (4) | 0.33010 (14) | 0.0291 (8) | |
C2 | −0.1697 (3) | 0.1479 (5) | 0.27017 (16) | 0.0396 (9) | |
H2A | −0.1744 | 0.0463 | 0.2880 | 0.048* | |
H2B | −0.1786 | 0.1282 | 0.2324 | 0.048* | |
C3 | −0.2681 (4) | 0.2508 (5) | 0.28404 (17) | 0.0472 (10) | |
H3A | −0.3435 | 0.1979 | 0.2741 | 0.057* | |
H3B | −0.2612 | 0.2670 | 0.3220 | 0.057* | |
C4 | −0.0090 (4) | 0.3239 (6) | 0.24694 (16) | 0.0473 (11) | |
H4A | 0.0029 | 0.4277 | 0.2624 | 0.071* | |
H4B | −0.0650 | 0.3308 | 0.2161 | 0.071* | |
H4C | 0.0656 | 0.2837 | 0.2372 | 0.071* | |
C5 | 0.1526 (3) | 0.3476 (4) | 0.51757 (14) | 0.0293 (8) | |
C6 | 0.0441 (3) | 0.4690 (4) | 0.58335 (14) | 0.0329 (8) | |
H6A | −0.0271 | 0.4303 | 0.5628 | 0.040* | |
H6B | 0.0304 | 0.5793 | 0.5924 | 0.040* | |
C7 | 0.0636 (3) | 0.3757 (4) | 0.63208 (14) | 0.0348 (8) | |
H7A | −0.0042 | 0.3895 | 0.6524 | 0.042* | |
H7B | 0.0682 | 0.2638 | 0.6230 | 0.042* | |
C8 | 0.2286 (4) | 0.5857 (5) | 0.55967 (17) | 0.0424 (10) | |
H8A | 0.2938 | 0.5502 | 0.5840 | 0.064* | |
H8B | 0.1926 | 0.6778 | 0.5739 | 0.064* | |
H8C | 0.2577 | 0.6125 | 0.5265 | 0.064* | |
C9 | 0.3348 (3) | −0.0675 (4) | 0.46063 (15) | 0.0366 (9) | |
H9 | 0.3551 | 0.0079 | 0.4868 | 0.044* | |
C10 | 0.4029 (3) | −0.1992 (5) | 0.45873 (15) | 0.0364 (9) | |
H10 | 0.4690 | −0.2131 | 0.4832 | 0.044* | |
C11 | 0.3752 (3) | −0.3110 (4) | 0.42118 (14) | 0.0320 (8) | |
C12 | 0.2771 (4) | −0.2850 (6) | 0.38681 (19) | 0.0553 (13) | |
H12 | 0.2538 | −0.3591 | 0.3606 | 0.066* | |
C13 | 0.2146 (4) | −0.1507 (5) | 0.39140 (19) | 0.0557 (13) | |
H13 | 0.1477 | −0.1343 | 0.3675 | 0.067* | |
C14 | 0.4440 (3) | −0.4535 (4) | 0.41878 (15) | 0.0341 (8) | |
H14 | 0.5072 | −0.4724 | 0.4444 | 0.041* | |
C15 | 0.4595 (3) | −0.7935 (4) | 0.35868 (15) | 0.0328 (8) | |
H15 | 0.3919 | −0.7803 | 0.3350 | 0.039* | |
C16 | 0.5253 (3) | −0.9406 (4) | 0.35960 (14) | 0.0315 (8) | |
C17 | 0.6222 (3) | −0.9662 (4) | 0.39506 (15) | 0.0329 (8) | |
H17 | 0.6453 | −0.8902 | 0.4207 | 0.039* | |
C18 | 0.6836 (3) | −1.1017 (4) | 0.39256 (15) | 0.0349 (8) | |
H18 | 0.7498 | −1.1169 | 0.4169 | 0.042* | |
C19 | 0.5620 (4) | −1.1910 (5) | 0.32486 (18) | 0.0481 (11) | |
H19 | 0.5398 | −1.2707 | 0.3004 | 0.058* | |
C20 | 0.4943 (4) | −1.0576 (5) | 0.32386 (18) | 0.0447 (10) | |
H20 | 0.4281 | −1.0462 | 0.2993 | 0.054* | |
Atomic displacement parameters (Å2) top | U11 | U22 | U33 | U12 | U13 | U23 |
Zn | 0.0318 (3) | 0.0277 (3) | 0.0314 (3) | 0.00704 (16) | −0.00087 (18) | −0.00366 (16) |
S1 | 0.0302 (5) | 0.0343 (5) | 0.0365 (5) | 0.0010 (4) | 0.0022 (4) | −0.0003 (4) |
S2 | 0.0322 (5) | 0.0286 (5) | 0.0366 (5) | 0.0015 (4) | −0.0014 (4) | 0.0018 (4) |
S3 | 0.0317 (5) | 0.0317 (5) | 0.0360 (5) | −0.0020 (4) | 0.0025 (4) | −0.0042 (4) |
S4 | 0.0351 (5) | 0.0321 (5) | 0.0381 (5) | −0.0023 (4) | 0.0063 (4) | −0.0061 (4) |
O1 | 0.055 (2) | 0.0464 (18) | 0.0575 (19) | 0.0100 (15) | −0.0213 (15) | 0.0022 (15) |
O2 | 0.0492 (17) | 0.0422 (17) | 0.0404 (15) | 0.0154 (13) | −0.0074 (13) | −0.0085 (13) |
N1 | 0.0365 (17) | 0.0351 (17) | 0.0314 (16) | 0.0016 (14) | −0.0011 (13) | 0.0012 (13) |
N2 | 0.0322 (16) | 0.0243 (15) | 0.0347 (16) | 0.0031 (12) | 0.0012 (13) | −0.0011 (13) |
N3 | 0.0324 (16) | 0.0309 (16) | 0.0332 (16) | 0.0054 (13) | −0.0014 (13) | −0.0044 (13) |
N4 | 0.0312 (17) | 0.0334 (18) | 0.0484 (19) | 0.0091 (14) | 0.0029 (14) | −0.0042 (15) |
N5 | 0.0290 (16) | 0.0309 (17) | 0.0480 (19) | 0.0063 (13) | −0.0007 (14) | −0.0040 (15) |
N6 | 0.0402 (19) | 0.0330 (18) | 0.056 (2) | 0.0101 (15) | −0.0052 (16) | −0.0051 (16) |
C1 | 0.0294 (18) | 0.0252 (17) | 0.0321 (19) | 0.0043 (14) | 0.0007 (15) | −0.0046 (15) |
C2 | 0.036 (2) | 0.038 (2) | 0.042 (2) | −0.0015 (17) | −0.0091 (18) | −0.0033 (17) |
C3 | 0.037 (2) | 0.053 (3) | 0.049 (2) | −0.0003 (19) | −0.0100 (19) | 0.003 (2) |
C4 | 0.055 (3) | 0.055 (3) | 0.031 (2) | −0.002 (2) | 0.0009 (19) | 0.0096 (19) |
C5 | 0.0303 (19) | 0.0264 (18) | 0.0300 (18) | 0.0070 (14) | −0.0034 (15) | 0.0004 (14) |
C6 | 0.0304 (18) | 0.0319 (19) | 0.0366 (19) | 0.0075 (15) | 0.0035 (15) | −0.0026 (16) |
C7 | 0.043 (2) | 0.0253 (18) | 0.037 (2) | 0.0048 (16) | 0.0075 (17) | −0.0026 (16) |
C8 | 0.042 (2) | 0.033 (2) | 0.054 (2) | −0.0107 (17) | 0.0106 (19) | −0.0143 (19) |
C9 | 0.040 (2) | 0.033 (2) | 0.035 (2) | 0.0047 (17) | −0.0052 (17) | −0.0039 (16) |
C10 | 0.033 (2) | 0.033 (2) | 0.041 (2) | 0.0076 (16) | −0.0083 (17) | 0.0012 (17) |
C11 | 0.0304 (19) | 0.0296 (19) | 0.0359 (19) | 0.0018 (15) | 0.0028 (16) | −0.0006 (16) |
C12 | 0.049 (3) | 0.051 (3) | 0.060 (3) | 0.022 (2) | −0.022 (2) | −0.030 (2) |
C13 | 0.052 (3) | 0.049 (3) | 0.060 (3) | 0.025 (2) | −0.027 (2) | −0.021 (2) |
C14 | 0.0252 (18) | 0.033 (2) | 0.044 (2) | 0.0046 (15) | 0.0017 (16) | 0.0054 (17) |
C15 | 0.0259 (18) | 0.034 (2) | 0.038 (2) | 0.0058 (15) | 0.0007 (15) | 0.0005 (17) |
C16 | 0.0267 (18) | 0.0282 (19) | 0.040 (2) | 0.0012 (15) | 0.0032 (15) | −0.0001 (16) |
C17 | 0.0304 (19) | 0.0283 (19) | 0.039 (2) | −0.0001 (15) | 0.0001 (16) | −0.0018 (16) |
C18 | 0.0301 (19) | 0.034 (2) | 0.040 (2) | 0.0022 (16) | −0.0017 (16) | 0.0025 (17) |
C19 | 0.046 (2) | 0.038 (2) | 0.058 (3) | 0.0056 (19) | −0.007 (2) | −0.013 (2) |
C20 | 0.034 (2) | 0.038 (2) | 0.059 (3) | 0.0025 (17) | −0.0106 (19) | −0.010 (2) |
Geometric parameters (Å, º) top Zn—S1 | 2.4152 (12) | C4—H4A | 0.9800 |
Zn—S2 | 2.5152 (11) | C4—H4B | 0.9800 |
Zn—S3 | 2.3890 (12) | C4—H4C | 0.9800 |
Zn—S4 | 2.5162 (11) | C6—C7 | 1.495 (5) |
Zn—N3 | 2.068 (3) | C6—H6A | 0.9900 |
S1—C1 | 1.726 (4) | C6—H6B | 0.9900 |
S2—C1 | 1.705 (4) | C7—H7A | 0.9900 |
S3—C5 | 1.720 (4) | C7—H7B | 0.9900 |
S4—C5 | 1.711 (4) | C8—H8A | 0.9800 |
O1—C3 | 1.426 (5) | C8—H8B | 0.9800 |
O1—H1O | 0.841 (10) | C8—H8C | 0.9800 |
O2—C7 | 1.428 (5) | C9—C10 | 1.378 (5) |
O2—H2O | 0.838 (10) | C9—H9 | 0.9500 |
N1—C1 | 1.331 (5) | C10—C11 | 1.382 (5) |
N1—C4 | 1.468 (5) | C10—H10 | 0.9500 |
N1—C2 | 1.469 (5) | C11—C12 | 1.387 (5) |
N2—C5 | 1.331 (5) | C11—C14 | 1.460 (5) |
N2—C6 | 1.456 (5) | C12—C13 | 1.369 (6) |
N2—C8 | 1.461 (5) | C12—H12 | 0.9500 |
N3—C13 | 1.330 (5) | C13—H13 | 0.9500 |
N3—C9 | 1.337 (5) | C14—H14 | 0.9500 |
N4—C14 | 1.267 (5) | C15—C16 | 1.470 (5) |
N4—N5 | 1.405 (4) | C15—H15 | 0.9500 |
N5—C15 | 1.267 (5) | C16—C20 | 1.389 (5) |
N6—C19 | 1.329 (5) | C16—C17 | 1.393 (5) |
N6—C18 | 1.344 (5) | C17—C18 | 1.364 (5) |
C2—C3 | 1.506 (6) | C17—H17 | 0.9500 |
C2—H2A | 0.9900 | C18—H18 | 0.9500 |
C2—H2B | 0.9900 | C19—C20 | 1.383 (6) |
C3—H3A | 0.9900 | C19—H19 | 0.9500 |
C3—H3B | 0.9900 | C20—H20 | 0.9500 |
| | | |
N3—Zn—S3 | 117.15 (9) | N2—C6—H6A | 109.0 |
N3—Zn—S1 | 106.36 (9) | C7—C6—H6A | 109.0 |
S1—Zn—S3 | 136.48 (4) | N2—C6—H6B | 109.0 |
N3—Zn—S2 | 101.88 (9) | C7—C6—H6B | 109.0 |
S3—Zn—S2 | 96.05 (4) | H6A—C6—H6B | 107.8 |
S1—Zn—S2 | 73.10 (4) | O2—C7—C6 | 113.1 (3) |
N3—Zn—S4 | 102.55 (9) | O2—C7—H7A | 109.0 |
S3—Zn—S4 | 73.47 (4) | C6—C7—H7A | 109.0 |
S1—Zn—S4 | 99.01 (4) | O2—C7—H7B | 109.0 |
S2—Zn—S4 | 155.56 (4) | C6—C7—H7B | 109.0 |
C1—S1—Zn | 85.88 (13) | H7A—C7—H7B | 107.8 |
C1—S2—Zn | 83.17 (12) | N2—C8—H8A | 109.5 |
C5—S3—Zn | 85.07 (13) | N2—C8—H8B | 109.5 |
C5—S4—Zn | 81.33 (12) | H8A—C8—H8B | 109.5 |
C3—O1—H1O | 111 (4) | N2—C8—H8C | 109.5 |
C7—O2—H2O | 118 (4) | H8A—C8—H8C | 109.5 |
C1—N1—C4 | 120.9 (3) | H8B—C8—H8C | 109.5 |
C1—N1—C2 | 122.2 (3) | N3—C9—C10 | 122.5 (3) |
C4—N1—C2 | 116.9 (3) | N3—C9—H9 | 118.7 |
C5—N2—C6 | 122.3 (3) | C10—C9—H9 | 118.7 |
C5—N2—C8 | 121.5 (3) | C9—C10—C11 | 120.0 (3) |
C6—N2—C8 | 116.2 (3) | C9—C10—H10 | 120.0 |
C13—N3—C9 | 116.9 (3) | C11—C10—H10 | 120.0 |
C13—N3—Zn | 119.8 (3) | C10—C11—C12 | 117.4 (3) |
C9—N3—Zn | 123.3 (2) | C10—C11—C14 | 121.4 (3) |
C14—N4—N5 | 111.6 (3) | C12—C11—C14 | 121.2 (3) |
C15—N5—N4 | 112.1 (3) | C13—C12—C11 | 118.8 (4) |
C19—N6—C18 | 116.3 (3) | C13—C12—H12 | 120.6 |
N1—C1—S2 | 122.4 (3) | C11—C12—H12 | 120.6 |
N1—C1—S1 | 119.8 (3) | N3—C13—C12 | 124.4 (4) |
S2—C1—S1 | 117.8 (2) | N3—C13—H13 | 117.8 |
N1—C2—C3 | 112.3 (3) | C12—C13—H13 | 117.8 |
N1—C2—H2A | 109.2 | N4—C14—C11 | 120.9 (3) |
C3—C2—H2A | 109.2 | N4—C14—H14 | 119.5 |
N1—C2—H2B | 109.2 | C11—C14—H14 | 119.5 |
C3—C2—H2B | 109.2 | N5—C15—C16 | 119.9 (3) |
H2A—C2—H2B | 107.9 | N5—C15—H15 | 120.1 |
O1—C3—C2 | 112.1 (4) | C16—C15—H15 | 120.1 |
O1—C3—H3A | 109.2 | C20—C16—C17 | 117.7 (3) |
C2—C3—H3A | 109.2 | C20—C16—C15 | 120.7 (3) |
O1—C3—H3B | 109.2 | C17—C16—C15 | 121.6 (3) |
C2—C3—H3B | 109.2 | C18—C17—C16 | 119.2 (3) |
H3A—C3—H3B | 107.9 | C18—C17—H17 | 120.4 |
N1—C4—H4A | 109.5 | C16—C17—H17 | 120.4 |
N1—C4—H4B | 109.5 | N6—C18—C17 | 124.0 (3) |
H4A—C4—H4B | 109.5 | N6—C18—H18 | 118.0 |
N1—C4—H4C | 109.5 | C17—C18—H18 | 118.0 |
H4A—C4—H4C | 109.5 | N6—C19—C20 | 124.3 (4) |
H4B—C4—H4C | 109.5 | N6—C19—H19 | 117.8 |
N2—C5—S4 | 120.7 (3) | C20—C19—H19 | 117.8 |
N2—C5—S3 | 121.7 (3) | C19—C20—C16 | 118.5 (4) |
S4—C5—S3 | 117.7 (2) | C19—C20—H20 | 120.8 |
N2—C6—C7 | 113.0 (3) | C16—C20—H20 | 120.8 |
| | | |
C14—N4—N5—C15 | 170.1 (4) | Zn—N3—C9—C10 | −179.6 (3) |
C4—N1—C1—S2 | 178.3 (3) | N3—C9—C10—C11 | −0.2 (6) |
C2—N1—C1—S2 | −0.6 (5) | C9—C10—C11—C12 | −0.7 (6) |
C4—N1—C1—S1 | −0.1 (5) | C9—C10—C11—C14 | −178.6 (4) |
C2—N1—C1—S1 | −179.1 (3) | C10—C11—C12—C13 | 0.8 (7) |
Zn—S2—C1—N1 | −175.9 (3) | C14—C11—C12—C13 | 178.8 (5) |
Zn—S2—C1—S1 | 2.55 (18) | C9—N3—C13—C12 | −0.6 (8) |
Zn—S1—C1—N1 | 175.9 (3) | Zn—N3—C13—C12 | 179.8 (4) |
Zn—S1—C1—S2 | −2.64 (19) | C11—C12—C13—N3 | −0.2 (9) |
C1—N1—C2—C3 | 92.4 (4) | N5—N4—C14—C11 | −176.8 (3) |
C4—N1—C2—C3 | −86.6 (4) | C10—C11—C14—N4 | −177.2 (4) |
N1—C2—C3—O1 | 59.9 (4) | C12—C11—C14—N4 | 4.9 (6) |
C6—N2—C5—S4 | −179.6 (2) | N4—N5—C15—C16 | 179.5 (3) |
C8—N2—C5—S4 | 0.3 (5) | N5—C15—C16—C20 | −175.7 (4) |
C6—N2—C5—S3 | 2.3 (5) | N5—C15—C16—C17 | 2.4 (6) |
C8—N2—C5—S3 | −177.8 (3) | C20—C16—C17—C18 | 1.4 (6) |
Zn—S4—C5—N2 | −163.6 (3) | C15—C16—C17—C18 | −176.8 (3) |
Zn—S4—C5—S3 | 14.54 (17) | C19—N6—C18—C17 | −1.1 (6) |
Zn—S3—C5—N2 | 162.9 (3) | C16—C17—C18—N6 | −0.4 (6) |
Zn—S3—C5—S4 | −15.21 (18) | C18—N6—C19—C20 | 1.5 (7) |
C5—N2—C6—C7 | 86.1 (4) | N6—C19—C20—C16 | −0.5 (7) |
C8—N2—C6—C7 | −93.8 (4) | C17—C16—C20—C19 | −1.0 (6) |
N2—C6—C7—O2 | 56.2 (4) | C15—C16—C20—C19 | 177.2 (4) |
C13—N3—C9—C10 | 0.8 (6) | | |
Hydrogen-bond geometry (Å, º) topCg1 is the centroid of the Zn/S1/S2/C1 ring. |
D—H···A | D—H | H···A | D···A | D—H···A |
O1—H1O···O2i | 0.85 (5) | 1.92 (5) | 2.721 (5) | 158 (5) |
O2—H2O···N6ii | 0.84 (4) | 1.95 (4) | 2.769 (5) | 163 (5) |
C20—H20···O1iii | 0.95 | 2.32 | 3.233 (6) | 162 |
C6—H6B···Cg1i | 0.99 | 2.59 | 3.540 (4) | 162 |
Symmetry codes: (i) −x, −y+1, −z+1; (ii) −x+1, −y−1, −z+1; (iii) −x, y−3/2, −z+1/2. |
Summary of short inter-atomic contacts (Å) in (I) topContact | Distance | Symmetry operation |
H1O···H2O | 2.21 (7) | -x, 1 - y, 1 - z |
H4B···H13 | 2.30 | -x, 1/2 + y, 1/2 - z |
Zn···C6 | 3.835 (4) | -x, 1 - y, 1 - z |
Zn···H6B | 3.00 | -x, 1 - y, 1 - z |
C1···H6B | 2.88 | -x, 1 - y, 1 - z |
S1···H6B | 2.92 | -x, 1 - y, 1 - z |
S1···H15 | 2.98 | x, 1 + y, z |
S2···H7B | 2.89 | -x, -y, 1 - z |
S4···C14 | 3.217 (4) | x, 1 + y, z |
C2···H4A | 2.88 | -x, -1/2 + y, 1/2 - z |
C5···H18 | 2.77 | 1 - x, 1 - y, 1 - z |
C18···H2O | 2.89 (5) | 1 - x, 1 - y, 1 - z |
C19···H2O | 2.85 (4) | 1 - x, 1 - y, 1 - z |
N5···H8A | 2.73 | 1 - x, -y, 1 - z |
Percentage contributions of inter-atomic contacts to the Hirshfeld surfaces for (I) topContact | Percentage contribution |
H···H | 44.6 |
S···H/H···S | 15.4 |
C···H/H···C | 13.1 |
N···H/H···N | 10.2 |
O···H/H···O | 6.7 |
C···C | 2.8 |
S···N/N···S | 2.8 |
S···S | 1.5 |
C···S/S···C | 1.2 |
C···N/N···C | 1.0 |
Zn···H/H···Zn | 0.6 |
Zn···S/S···Zn | 0.1 |
Acknowledgements
We thank Sunway University for support of biological and crystal engineering studies of metal dithiocarbamates.
References
Addison, A. W., Rao, T. N., Reedijk, J., van Rijn, J. & Verschoor, G. C. (1984). J. Chem. Soc. Dalton Trans. pp. 1349–1356. CSD CrossRef Web of Science
Arman, H. D., Poplaukhin, P. & Tiekink, E. R. T. (2016). Acta Cryst. E72, 1234–1238. Web of Science CSD CrossRef IUCr Journals
Avila, V., Benson, R. E., Broker, G. A., Daniels, L. M. & Tiekink, E. R. T. (2006). Acta Cryst. E62, m1425–m1427. Web of Science CSD CrossRef IUCr Journals
Benson, R. E., Ellis, C. A., Lewis, C. E. & Tiekink, E. R. T. (2007). CrystEngComm, 9, 930–940. Web of Science CSD CrossRef CAS
Berdugo, E. & Tiekink, E. R. T. (2009). Acta Cryst. E65, m1444–m1445. Web of Science CSD CrossRef IUCr Journals
Brandenburg, K. (2006). DIAMOND. Crystal Impact GbR, Bonn, Germany.
Broker, G. A. & Tiekink, E. R. T. (2011). Acta Cryst. E67, m320–m321. Web of Science CSD CrossRef CAS IUCr Journals
Chai, J., Lai, C. S., Yan, J. & Tiekink, E. R. T. (2003). Appl. Organomet. Chem. 17, 249–250. Web of Science CSD CrossRef CAS
Fan, J., Wei, F.-X., Zhang, W.-G., Yin, X., Lai, C. S. & Tiekink, E. R. T. (2007). Acta Chim. Sinica, 65, 2014–2018. CAS
Farrugia, L. J. (2012). J. Appl. Cryst. 45, 849–854. Web of Science CrossRef CAS IUCr Journals
Groom, C. R., Bruno, I. J., Lightfoot, M. P. & Ward, S. C. (2016). Acta Cryst. B72, 171–179. Web of Science CSD CrossRef IUCr Journals
Higashi, T. (1995). ABSCOR. Rigaku Corporation, Tokyo, Japan.
Howie, R. A., de Lima, G. M., Menezes, D. C., Wardell, J. L., Wardell, S. M. S. V., Young, D. J. & Tiekink, E. R. T. (2008). CrystEngComm, 10, 1626–1637. Web of Science CSD CrossRef CAS
Jotani, M. M., Poplaukhin, P., Arman, H. D. & Tiekink, E. R. T. (2016). Acta Cryst. E72, 1085–1092. Web of Science CSD CrossRef IUCr Journals
Jotani, M. M., Poplaukhin, P., Arman, H. D. & Tiekink, E. R. T. (2017). Z. Kristallogr. 232, 287–298. CAS
Klevtsova, R. F., Glinskaya, L. A., Berus, E. I. & Larionov, S. V. (2001). J. Struct. Chem. 42, 639–647. Web of Science CSD CrossRef CAS
Lai, C. S. & Tiekink, E. R. T. (2003). Appl. Organomet. Chem. 17, 251–252. Web of Science CSD CrossRef CAS
Lai, C. S. & Tiekink, E. R. T. (2006). Z. Kristallogr. 221, 288–293. Web of Science CSD CrossRef CAS
McKinnon, J. J., Jayatilaka, D. & Spackman, M. A. (2007). Chem. Commun. pp. 3814–3816. Web of Science CrossRef
Molecular Structure Corporation & Rigaku (2005). CrystalClear. MSC, The Woodlands, Texas, USA, and Rigaku Corporation, Tokyo, Japan.
Poplaukhin, P., Arman, H. D. & Tiekink, E. R. T. (2012). Z. Kristallogr. 227, 363–368. Web of Science CSD CrossRef CAS
Poplaukhin, P. & Tiekink, E. R. T. (2010). CrystEngComm, 12, 1302–1306. Web of Science CSD CrossRef
Sheldrick, G. M. (2008). Acta Cryst. A64, 112–122. Web of Science CrossRef CAS IUCr Journals
Sheldrick, G. M. (2015). Acta Cryst. C71, 3–8. Web of Science CrossRef IUCr Journals
Shoshnik, R., Elengoz, H. & Goldberg, I. (2005). Acta Cryst. C61, m187–m189. Web of Science CSD CrossRef CAS IUCr Journals
Tiekink, E. R. T. (2003). CrystEngComm, 5, 101–113. Web of Science CrossRef CAS
Tiekink, E. R. T. (2017). Coord. Chem. Rev. 345, 209–228. Web of Science CrossRef CAS
Westrip, S. P. (2010). J. Appl. Cryst. 43, 920–925. Web of Science CrossRef CAS IUCr Journals
This is an open-access article distributed under the terms of the Creative Commons Attribution (CC-BY) Licence, which permits unrestricted use, distribution, and reproduction in any medium, provided the original authors and source are cited.
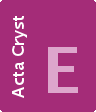 | CRYSTALLOGRAPHIC COMMUNICATIONS |
ISSN: 2056-9890
Open

access