1. Chemical context
Compounds expressed as C6H5—C(=X)—Y—CH2—CH2—Y—C(=X)—C6H5 (X, Y = O or S) can be considered to be monomeric models for polymers, [—C(=X)—C6H4—C(=X)—Y—CH2—CH2—Y—]x, namely, X = Y = O, poly(ethylene terephthalate) (designated herein as polymer A); X = O and Y = S, poly(ethylene dithioterephthalate) (polymer B); X = Y = S, poly(ethylene tetrathioterephthalate) (polymer C); X = S and Y = O, poly(ethylene dithionoterephthalate) (polymer D). It is well established that the solution, mechanical and thermal properties of such aromatic polymers are essentially determined by the conformational characteristics of the Y—CH2—CH2—Y unit (referred hereafter to as the spacer) and intermolecular interactions between the benzene rings (Sasanuma, 2009
; Sasanuma et al., 2013
). In expectation that replacement of oxygen by sulfur at the X or Y site would affect the spacer conformation, and π–π and C—H⋯π interactions of the benzene rings, and thus lead to variations in the physical properties, we synthesized polymers B and C, and characterized them by X-ray diffraction, NMR spectroscopy, thermal analyses, molecular orbital calculations and statistical mechanics of the chain molecules (Abe & Sasanuma, 2012
). Herein, the monomeric models for polymers A–D are termed models A–D, respectively.
By molecular orbital calculations at the second-order Møller–Plesset perturbation (MP2) level with moderate-size basis sets, we have determined the most stable conformations of the Y—CH2—CH2—Y parts of the models and evaluated their free energies relative to that of the all-trans form as follows: model A, tgt and −1.1 kcal mol−1 (Sasanuma, 2009
); model B, g±tg∓ and −3.1 kcal mol−1 (Abe & Sasanuma, 2012
); model C, g±tg∓ and −2.1 kcal mol−1 (Abe & Sasanuma, 2012
); model D, tgt and −1.7 kcal mol−1 (this study). We have also predicted that an asymmetric model compound, C6H5—C(=O)—O—CH2—CH2—O—C(=S)—C6H5 (model E), would be most stabilized in the tg±g∓ conformation with a free energy of −1.8 kcal mol−1 (this study). However, not all the models and polymers crystallize in the lowest-energy conformations: model (polymer) A, ttt (ttt) (Pérez & Brisse, 1976
; Daubeny et al., 1954
); model (polymer) B, g±tg∓ (g±tg∓) (Deguire & Brisse, 1988
; Abe & Sasanuma, 2012
); model (polymer) C, g±tg∓ (amorphous) (Abe et al., 2011
; Abe & Sasanuma, 2012
). In the crystals, the molecules adopt conformations so as to form intermolecular interactions effectively and minimize the total of intramolecular and intermolecular interaction energies. Interestingly, however, models A–C crystallize in the same spacer conformation as those of the corresponding polymers; therefore, the crystal structure of the model suggests the polymer conformation. This study has aimed to determine crystal structures of the title compounds (models D and E) to predict the crystal conformations of polymers D and E on the above hypothesis.
2. Structural commentary
The molecule of model D lies on an inversion centre and the asymmetric unit contains one half-molecule. The central O—CH2—CH2—O unit adopts an all-trans conformation (Fig. 1
). The molecule of model E is also located on an inversion centre and the O—CH2—CH2—O bond sequence is in an all-trans conformation. Since the molecule has carbonyl and thiocarbonyl groups, the atoms S and O (S1 and O2) are each assumed to be disordered over two equivalent sites about the inversion centre with equal occupancies (Fig. 2
). Consequently, it was proved that all the models (A, D and E) with the O—CH2—CH2—O spacer crystallize with all-trans structures, although models A, D and E in the free state are most stabilized in tgt, tgt, and tg±g∓ conformations, respectively.
| Figure 1 The molecular structure of model D, showing atom-labelling scheme. Displacement ellipsoids are drawn at the 50% probability level. The unlabelled atoms are related to the labelled atoms by inversion symmetry (symmetry code: 2 − x, 2 − y, 2 − z,). H atoms are represented by spheres of arbitrary size. |
| Figure 2 The molecular structure of model E, showing atom-labelling scheme. Displacement ellipsoids are drawn at the 50% probability level. The unlabelled atoms are related to the labelled atoms by inversion symmetry (symmetry code: 2 − x, 2 − y, 2 − z,). H atoms are represented by spheres of arbitrary size. |
3. Supramolecular features
The compounds of models D and E are isotypic and crystallize in the space group P21/c. There are no classical hydrogen bonds but intermolecular close contacts between atoms C and S [C1—S1i = 3.391 (3) and 3.308 (3) Å for models D and E, respectively; symmetry code: (i) x, –y +
, z –
]. Both compounds also have C—H⋯π interactions (Tables 1
and 2
) and form layer structures parallel to the bc plane via these intermolecular interactions (Figs. 3
and 4
).
D—H⋯A | D—H | H⋯A | D⋯A | D—H⋯A | C3—H3⋯Cg1i | 0.95 | 2.92 | 3.721 (3) | 143 | Symmetry code: (i) x, −y + , z − . | |
D—H⋯A | D—H | H⋯A | D⋯A | D—H⋯A | C3—H3⋯Cg1i | 0.95 | 2.89 | 3.641 (3) | 137 | Symmetry code: (i) x, −y + , z − . | |
| Figure 3 A packing diagram of model D, showing intermolecular C⋯S contacts (red dotted lines) and C—H⋯π interactions (blue dotted lines). |
| Figure 4 A packing diagram of model E, showing intermolecular C⋯S contacts (red dotted lines) and C—H⋯π interactions (blue dotted lines). |
3.1. Synthesis and crystallization
Benzoyl chloride (10.0 ml, 87 mmol) was added dropwise under a nitrogen atmosphere to ethylene glycol (2.4 ml, 43 mmol) and pyridine (7.0 ml, 87 mmol) placed in a four-necked flask connected to a drying tube filled with calcium chloride, and the mixture was stirred at room temperature overnight. Water was added to the reaction mixture to yield a precipitate, which was collected by filtration, dissolved in chloroform, washed thrice with 5% aqueous solution of sodium bicarbonate, and dried over anhydrous magnesium sulfate overnight. The liquid phase was separated by filtration and condensed on a rotary evaporator, and the residue was recrystallized from ethanol (15 ml). The white crystallites thus obtained were dried under reduced pressure at room temperature overnight to yield ethane-1,2-diyl dibenzoate (8.3 g, 71%). The synthesized ethane-1,2-diyl dibenzoate (0.10 g, 0.37 mmol) was ground in a mortar and mixed thoroughly with Lawesson's reagent (0.24 g, 0.59 mmol), and the powder mixture was moved to a 15 ml vial container and placed in a Yuasa PRE-7017R microwave oven. The powder was heated under the following microwave irradiation at 500 W: on for 2.0 min – off for several seconds – on for 1.0 min. The above handling was repeated ten times to obtain the product sufficiently.
The crude product was extracted with chloroform and condensed under reduced pressure. The residue was dissolved in a mixed solvent of ethyl acetate and n-hexane (1:9 v/v) and subjected to column chromatography. The yellowish fraction (Rf = 0.5) was collected and condensed, and the residue underwent column chromatography again with a mixed solvent of toluene and n-hexane (1:5 v/v). Two yellow fractions [(1) Rf = 0.1 and (2) 0.3 − 0.5] were stratified and collected separately. The layer (1) was condensed and recrystallized from ethanol to yield a yellow solid, which was identified as 2-(benzenecarbothioyloxy)ethyl benzoate (model E, yield 23%) by 1H and 13C NMR, and the layer (2) was condensed and dried at room temperature overnight to yield a red solid, which was identified as 2-(benzenecarbothioyloxy)ethyl benzenecarbothioate (model D, yield 0.9%). A small quantity of model D was dissolved in chloroform in a thin vial container. The vessel was placed in a larger vial containing a small amount of n-hexane, and the outer container was capped. After a week, single crystals were found to be formed in the inner vessel. Single crystals of model E were prepared similarly.
4. Refinement
Crystal data, data collection and structure refinement details are summarized in Table 3
. All H atoms were geometrically positioned with C—H = 0.95 and 0.99 Å for the aromatic and methylene groups, respectively, and were refined as riding with Uiso(H) = 1.2 Ueq(C).
| model D | model E | Crystal data | Chemical formula | C16H14O2S2 | C16H14O3S | Mr | 302.39 | 286.33 | Crystal system, space group | Monoclinic, P21/c | Monoclinic, P21/c | Temperature (K) | 173 | 173 | a, b, c (Å) | 8.829 (5), 11.680 (7), 7.727 (5) | 8.800 (5), 11.403 (6), 7.506 (4) | β (°) | 113.475 (10) | 113.831 (6) | V (Å3) | 730.9 (8) | 689.0 (6) | Z | 2 | 2 | Radiation type | Mo Kα | Mo Kα | μ (mm−1) | 0.36 | 0.24 | Crystal size (mm) | 0.40 × 0.40 × 0.20 | 0.40 × 0.40 × 0.10 | | Data collection | Diffractometer | Bruker APEXII CCD area detector | Bruker APEXII CCD area detector | Absorption correction | Multi-scan (SADABS; Sheldrick, 1996 ) | Multi-scan (SADABS; Sheldrick, 1996 ) | Tmin, Tmax | 0.88, 0.93 | 0.84, 0.98 | No. of measured, independent and observed [I > 2σ(I)] reflections | 4017, 1617, 1487 | 3265, 1523, 1335 | Rint | 0.014 | 0.015 | (sin θ/λ)max (Å−1) | 0.648 | 0.653 | | Refinement | R[F2 > 2σ(F2)], wR(F2), S | 0.029, 0.080, 1.03 | 0.038, 0.090, 1.08 | No. of reflections | 1617 | 1523 | No. of parameters | 91 | 100 | H-atom treatment | H-atom parameters constrained | H-atom parameters constrained | Δρmax, Δρmin (e Å−3) | 0.39, −0.20 | 0.19, −0.16 | Computer programs: APEX2, SAINT, XSHEL and XCIF (Bruker, 2012 ) SHELXS97 (Sheldrick, 2008 ) and SHELXL2013 (Sheldrick, 2015 ). | |
Supporting information
For both structures, data collection: APEX2 (Bruker, 2012); cell refinement: SAINT (Bruker, 2012). Data reduction: SAINT for model_D; SAINT (Bruker, 2012) for model_E. For both structures, program(s) used to solve structure: SHELXS97 (Sheldrick, 2008); program(s) used to refine structure: SHELXL2013 (Sheldrick, 2015); molecular graphics: XSHEL (Bruker, 2012); software used to prepare material for publication: XCIF (Bruker, 2012).
2-(Benzenecarbothioyloxy)ethyl benzenecarbothioate (model_D)
top Crystal data top C16H14O2S2 | F(000) = 316 |
Mr = 302.39 | Dx = 1.374 Mg m−3 |
Monoclinic, P21/c | Mo Kα radiation, λ = 0.71073 Å |
a = 8.829 (5) Å | Cell parameters from 2339 reflections |
b = 11.680 (7) Å | θ = 2.5–27.6° |
c = 7.727 (5) Å | µ = 0.36 mm−1 |
β = 113.475 (10)° | T = 173 K |
V = 730.9 (8) Å3 | Prismatic, yellow |
Z = 2 | 0.40 × 0.40 × 0.20 mm |
Data collection top Bruker APEXII CCD area detector diffractometer | 1617 independent reflections |
Radiation source: fine-focus sealed tube | 1487 reflections with I > 2σ(I) |
Graphite monochromator | Rint = 0.014 |
Detector resolution: 8.3333 pixels mm-1 | θmax = 27.4°, θmin = 2.5° |
φ and ω scans | h = −10→11 |
Absorption correction: multi-scan (SADABS; Sheldrick, 1996) | k = −13→15 |
Tmin = 0.88, Tmax = 0.93 | l = −9→5 |
4017 measured reflections | |
Refinement top Refinement on F2 | 0 restraints |
Least-squares matrix: full | Hydrogen site location: inferred from neighbouring sites |
R[F2 > 2σ(F2)] = 0.029 | H-atom parameters constrained |
wR(F2) = 0.080 | w = 1/[σ2(Fo2) + (0.0425P)2 + 0.2706P] where P = (Fo2 + 2Fc2)/3 |
S = 1.03 | (Δ/σ)max = 0.001 |
1617 reflections | Δρmax = 0.39 e Å−3 |
91 parameters | Δρmin = −0.20 e Å−3 |
Special details top Experimental. SADABS (Sheldrick 1996) |
Geometry. All esds (except the esd in the dihedral angle between two l.s. planes) are estimated using the full covariance matrix. The cell esds are taken into account individually in the estimation of esds in distances, angles and torsion angles; correlations between esds in cell parameters are only used when they are defined by crystal symmetry. An approximate (isotropic) treatment of cell esds is used for estimating esds involving l.s. planes. |
Fractional atomic coordinates and isotropic or equivalent isotropic displacement parameters (Å2) top | x | y | z | Uiso*/Ueq | |
C1 | 0.70662 (15) | 0.86862 (11) | 0.98706 (17) | 0.0209 (3) | |
C2 | 0.52641 (15) | 0.87852 (10) | 0.86705 (17) | 0.0205 (3) | |
C3 | 0.41444 (16) | 0.80285 (12) | 0.89460 (19) | 0.0271 (3) | |
H3 | 0.4532 | 0.7468 | 0.9916 | 0.033* | |
C4 | 0.24591 (17) | 0.80973 (13) | 0.7796 (2) | 0.0312 (3) | |
H4 | 0.1705 | 0.7586 | 0.7991 | 0.037* | |
C5 | 0.18837 (16) | 0.89124 (13) | 0.6367 (2) | 0.0309 (3) | |
H5 | 0.0741 | 0.8947 | 0.5572 | 0.037* | |
C6 | 0.29824 (17) | 0.96779 (13) | 0.6101 (2) | 0.0316 (3) | |
H6 | 0.2585 | 1.024 | 0.5136 | 0.038* | |
C7 | 0.46690 (16) | 0.96209 (11) | 0.72503 (19) | 0.0259 (3) | |
H7 | 0.5413 | 1.0148 | 0.707 | 0.031* | |
C8 | 0.97120 (15) | 0.94978 (12) | 1.04144 (19) | 0.0267 (3) | |
H8A | 1.0184 | 0.8771 | 1.0194 | 0.032* | |
H8B | 1.0041 | 0.9599 | 1.1788 | 0.032* | |
O1 | 0.79324 (11) | 0.95006 (8) | 0.94319 (13) | 0.0252 (2) | |
S1 | 0.79118 (4) | 0.77062 (3) | 1.15081 (5) | 0.02945 (13) | |
Atomic displacement parameters (Å2) top | U11 | U22 | U33 | U12 | U13 | U23 |
C1 | 0.0204 (6) | 0.0227 (6) | 0.0205 (6) | −0.0032 (5) | 0.0091 (5) | −0.0036 (5) |
C2 | 0.0189 (6) | 0.0224 (6) | 0.0205 (6) | −0.0013 (5) | 0.0080 (5) | −0.0036 (5) |
C3 | 0.0232 (6) | 0.0300 (7) | 0.0265 (7) | −0.0040 (5) | 0.0081 (5) | 0.0023 (5) |
C4 | 0.0221 (6) | 0.0373 (8) | 0.0334 (7) | −0.0072 (6) | 0.0103 (6) | −0.0024 (6) |
C5 | 0.0191 (6) | 0.0376 (8) | 0.0318 (7) | 0.0020 (5) | 0.0057 (5) | −0.0050 (6) |
C6 | 0.0274 (7) | 0.0315 (7) | 0.0314 (7) | 0.0051 (6) | 0.0068 (6) | 0.0049 (6) |
C7 | 0.0244 (7) | 0.0244 (6) | 0.0283 (7) | −0.0010 (5) | 0.0099 (5) | 0.0014 (5) |
C8 | 0.0170 (6) | 0.0314 (7) | 0.0281 (6) | −0.0041 (5) | 0.0051 (5) | 0.0030 (5) |
O1 | 0.0174 (4) | 0.0269 (5) | 0.0282 (5) | −0.0042 (3) | 0.0057 (4) | 0.0037 (4) |
S1 | 0.02236 (19) | 0.0332 (2) | 0.0302 (2) | −0.00007 (13) | 0.00763 (14) | 0.00936 (13) |
Geometric parameters (Å, º) top C1—O1 | 1.3458 (16) | C5—C6 | 1.393 (2) |
C1—C2 | 1.4931 (18) | C5—H5 | 0.95 |
C1—S1 | 1.6481 (14) | C6—C7 | 1.399 (2) |
C2—C3 | 1.4042 (19) | C6—H6 | 0.95 |
C2—C7 | 1.4049 (19) | C7—H7 | 0.95 |
C3—C4 | 1.399 (2) | C8—O1 | 1.4474 (17) |
C3—H3 | 0.95 | C8—C8i | 1.518 (3) |
C4—C5 | 1.392 (2) | C8—H8A | 0.99 |
C4—H4 | 0.95 | C8—H8B | 0.99 |
| | | |
O1—C1—C2 | 111.15 (11) | C6—C5—H5 | 120.0 |
O1—C1—S1 | 123.72 (10) | C5—C6—C7 | 120.22 (13) |
C2—C1—S1 | 125.13 (9) | C5—C6—H6 | 119.9 |
C3—C2—C7 | 119.27 (12) | C7—C6—H6 | 119.9 |
C3—C2—C1 | 119.84 (12) | C6—C7—C2 | 120.09 (12) |
C7—C2—C1 | 120.89 (11) | C6—C7—H7 | 120.0 |
C4—C3—C2 | 120.17 (13) | C2—C7—H7 | 120.0 |
C4—C3—H3 | 119.9 | O1—C8—C8i | 104.66 (13) |
C2—C3—H3 | 119.9 | O1—C8—H8A | 110.8 |
C5—C4—C3 | 120.21 (13) | C8i—C8—H8A | 110.8 |
C5—C4—H4 | 119.9 | O1—C8—H8B | 110.8 |
C3—C4—H4 | 119.9 | C8i—C8—H8B | 110.8 |
C4—C5—C6 | 120.02 (13) | H8A—C8—H8B | 108.9 |
C4—C5—H5 | 120.0 | C1—O1—C8 | 118.26 (10) |
Symmetry code: (i) −x+2, −y+2, −z+2. |
2-(Benzenecarbothioyloxy)ethyl benzoate (model_E)
top Crystal data top C16H14O3S | F(000) = 300 |
Mr = 286.33 | Dx = 1.380 Mg m−3 |
Monoclinic, P21/c | Mo Kα radiation, λ = 0.71073 Å |
a = 8.800 (5) Å | Cell parameters from 1991 reflections |
b = 11.403 (6) Å | θ = 2.5–27.6° |
c = 7.506 (4) Å | µ = 0.24 mm−1 |
β = 113.831 (6)° | T = 173 K |
V = 689.0 (6) Å3 | Prismatic, yellow |
Z = 2 | 0.40 × 0.40 × 0.10 mm |
Data collection top Bruker APEXII CCD area detector diffractometer | 1523 independent reflections |
Radiation source: fine-focus sealed tube | 1335 reflections with I > 2σ(I) |
Graphite monochromator | Rint = 0.015 |
Detector resolution: 8.3333 pixels mm-1 | θmax = 27.7°, θmin = 2.5° |
φ and ω scans | h = −11→11 |
Absorption correction: multi-scan (SADABS; Sheldrick, 1996) | k = −6→14 |
Tmin = 0.84, Tmax = 0.98 | l = −8→9 |
3265 measured reflections | |
Refinement top Refinement on F2 | 0 restraints |
Least-squares matrix: full | Hydrogen site location: inferred from neighbouring sites |
R[F2 > 2σ(F2)] = 0.038 | H-atom parameters constrained |
wR(F2) = 0.090 | w = 1/[σ2(Fo2) + (0.0288P)2 + 0.2342P] where P = (Fo2 + 2Fc2)/3 |
S = 1.08 | (Δ/σ)max = 0.002 |
1523 reflections | Δρmax = 0.19 e Å−3 |
100 parameters | Δρmin = −0.16 e Å−3 |
Special details top Experimental. SADABS (Sheldrick 1996) |
Geometry. All esds (except the esd in the dihedral angle between two l.s. planes) are estimated using the full covariance matrix. The cell esds are taken into account individually in the estimation of esds in distances, angles and torsion angles; correlations between esds in cell parameters are only used when they are defined by crystal symmetry. An approximate (isotropic) treatment of cell esds is used for estimating esds involving l.s. planes. |
Fractional atomic coordinates and isotropic or equivalent isotropic displacement parameters (Å2) top | x | y | z | Uiso*/Ueq | Occ. (<1) |
S1 | 0.78695 (19) | 0.76827 (18) | 1.1323 (3) | 0.0410 (3) | 0.5 |
O2 | 0.7837 (8) | 0.7828 (6) | 1.0959 (10) | 0.087 (3) | 0.5 |
O1 | 0.79258 (12) | 0.94978 (9) | 0.93503 (15) | 0.0404 (3) | |
C1 | 0.70747 (18) | 0.86474 (13) | 0.9769 (2) | 0.0372 (3) | |
C2 | 0.52662 (17) | 0.87657 (12) | 0.86227 (19) | 0.0338 (3) | |
C3 | 0.4186 (2) | 0.79742 (14) | 0.8908 (2) | 0.0428 (4) | |
H3 | 0.461 | 0.7372 | 0.9857 | 0.051* | |
C4 | 0.2497 (2) | 0.80604 (15) | 0.7815 (2) | 0.0486 (4) | |
H4 | 0.1762 | 0.7516 | 0.8014 | 0.058* | |
C5 | 0.1873 (2) | 0.89288 (16) | 0.6442 (3) | 0.0492 (4) | |
H5 | 0.0711 | 0.8978 | 0.5681 | 0.059* | |
C6 | 0.2934 (2) | 0.97304 (15) | 0.6168 (2) | 0.0470 (4) | |
H6 | 0.2498 | 1.0336 | 0.5229 | 0.056* | |
C7 | 0.46254 (18) | 0.96570 (12) | 0.7252 (2) | 0.0381 (3) | |
H7 | 0.5351 | 1.0213 | 0.7064 | 0.046* | |
C8 | 0.97020 (18) | 0.94635 (14) | 1.0342 (2) | 0.0440 (4) | |
H8A | 1.0153 | 0.8744 | 0.9999 | 0.053* | |
H8B | 1.0047 | 0.9485 | 1.1771 | 0.053* | |
Atomic displacement parameters (Å2) top | U11 | U22 | U33 | U12 | U13 | U23 |
S1 | 0.0345 (5) | 0.0398 (5) | 0.0452 (6) | 0.0040 (4) | 0.0125 (4) | 0.0086 (5) |
O2 | 0.094 (4) | 0.073 (4) | 0.086 (5) | −0.007 (3) | 0.028 (3) | 0.007 (3) |
O1 | 0.0368 (5) | 0.0394 (6) | 0.0392 (6) | −0.0059 (4) | 0.0093 (4) | 0.0043 (4) |
C1 | 0.0457 (8) | 0.0330 (7) | 0.0324 (8) | −0.0042 (6) | 0.0153 (6) | −0.0013 (6) |
C2 | 0.0419 (8) | 0.0332 (7) | 0.0270 (7) | −0.0048 (6) | 0.0144 (6) | −0.0058 (5) |
C3 | 0.0536 (9) | 0.0417 (8) | 0.0333 (8) | −0.0113 (7) | 0.0176 (7) | −0.0028 (6) |
C4 | 0.0475 (9) | 0.0563 (10) | 0.0462 (10) | −0.0191 (8) | 0.0233 (7) | −0.0124 (8) |
C5 | 0.0380 (8) | 0.0592 (10) | 0.0489 (10) | −0.0037 (7) | 0.0159 (7) | −0.0100 (8) |
C6 | 0.0445 (8) | 0.0470 (9) | 0.0464 (10) | 0.0036 (7) | 0.0151 (7) | 0.0021 (7) |
C7 | 0.0427 (8) | 0.0343 (7) | 0.0380 (8) | −0.0027 (6) | 0.0171 (6) | −0.0010 (6) |
C8 | 0.0365 (8) | 0.0462 (9) | 0.0437 (9) | −0.0044 (7) | 0.0103 (6) | 0.0033 (7) |
Geometric parameters (Å, º) top S1—C1 | 1.549 (3) | C4—H4 | 0.95 |
O2—C1 | 1.279 (6) | C5—C6 | 1.380 (2) |
O1—C1 | 1.3381 (18) | C5—H5 | 0.95 |
O1—C8 | 1.4349 (19) | C6—C7 | 1.380 (2) |
C1—C2 | 1.478 (2) | C6—H6 | 0.95 |
C2—C3 | 1.389 (2) | C7—H7 | 0.95 |
C2—C7 | 1.393 (2) | C8—C8i | 1.501 (3) |
C3—C4 | 1.381 (2) | C8—H8A | 0.99 |
C3—H3 | 0.95 | C8—H8B | 0.99 |
C4—C5 | 1.374 (3) | | |
| | | |
C1—O1—C8 | 117.01 (12) | C4—C5—C6 | 119.98 (15) |
O2—C1—O1 | 120.5 (3) | C4—C5—H5 | 120.0 |
O2—C1—C2 | 127.6 (3) | C6—C5—H5 | 120.0 |
O1—C1—C2 | 111.73 (13) | C5—C6—C7 | 120.36 (16) |
O1—C1—S1 | 124.48 (13) | C5—C6—H6 | 119.8 |
C2—C1—S1 | 123.75 (13) | C7—C6—H6 | 119.8 |
C3—C2—C7 | 119.33 (14) | C6—C7—C2 | 119.85 (14) |
C3—C2—C1 | 119.61 (14) | C6—C7—H7 | 120.1 |
C7—C2—C1 | 121.06 (13) | C2—C7—H7 | 120.1 |
C4—C3—C2 | 120.12 (15) | O1—C8—C8i | 104.96 (15) |
C4—C3—H3 | 119.9 | O1—C8—H8A | 110.8 |
C2—C3—H3 | 119.9 | C8i—C8—H8A | 110.8 |
C5—C4—C3 | 120.33 (15) | O1—C8—H8B | 110.8 |
C5—C4—H4 | 119.8 | C8i—C8—H8B | 110.8 |
C3—C4—H4 | 119.8 | H8A—C8—H8B | 108.8 |
Symmetry code: (i) −x+2, −y+2, −z+2. |
C—H···π interaction geometry (Å, °) for (model_D) topCg1 is the centroid of the C2–C7 ring. |
D—H···A | D—H | H···A | D···A | D—H···A |
C3—H3···Cg1i | 0.95 | 2.92 | 3.721 (3) | 143 |
Symmetry code: (i) x, -y + 1/2, z - 1/2. |
C—H···π interaction geometry (Å, °) for (model_E) topCg1 is the centroid of the C2–C7 ring. |
D—H···A | D—H | H···A | D···A | D—H···A |
C3—H3···Cg1i | 0.95 | 2.89 | 3.641 (3) | 137 |
Symmetry code: (i) x, -y + 1/2, z - 1/2. |
Funding information
This study was partially supported by the Grants-in-Aid for Scientific Research (C) (16 K05906) from the Japan Society for the Promotion of Science.
References
Daubeny, R. de P., Bunn, C. W. & Brown, C. J. (1954). Proc. R. Soc. London Ser. A, 226, 531–542. CAS
Abe, D. & Sasanuma, Y. (2012). Polym. Chem. 3, 1576–1587. Web of Science CrossRef CAS
Abe, D., Sasanuma, Y. & Sato, H. (2011). Acta Cryst. E67, o961. Web of Science CSD CrossRef IUCr Journals
Bruker (2012). APEX2, SAINT, XCIF and XSHEL. Bruker AXS Inc., Madison, Wisconsin, USA.
Deguire, S. & Brisse, F. (1988). Can. J. Chem. 66, 341–347. CSD CrossRef CAS Web of Science
Pérez, S. & Brisse, F. (1976). Acta Cryst. B32, 470–474. CSD CrossRef IUCr Journals Web of Science
Sasanuma, Y. (2009). Macromolecules, 42, 2854–2862. Web of Science CrossRef CAS
Sasanuma, Y., Wagai, Y., Suzuki, N. & Abe, D. (2013). Polymer, 54, 3904–3913. Web of Science CrossRef CAS
Sheldrick, G. M. (1996). SADABS. University of Göttingen, Germany.
Sheldrick, G. M. (2008). Acta Cryst. A64, 112–122. Web of Science CrossRef CAS IUCr Journals
Sheldrick, G. M. (2015). Acta Cryst. C71, 3–8. Web of Science CrossRef IUCr Journals
This is an open-access article distributed under the terms of the Creative Commons Attribution (CC-BY) Licence, which permits unrestricted use, distribution, and reproduction in any medium, provided the original authors and source are cited.
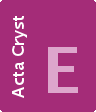 | CRYSTALLOGRAPHIC COMMUNICATIONS |
ISSN: 2056-9890
Open

access