1. Chemical context
Reducing carbohydrates, for instance aldoses (glucose, mannose, xylose) or ketoses (fructose, ribulose), mutarotate in solutions such that the predominant species in equilibrium consist of cyclic pyranose and furanose hemiacetals or hemiketals, respectively (Angyal, 1992
). Free aldehyde or ketone forms are thermodynamically unfavorable and normally comprise less than 1% of the population in the equilibria. Crystallization of reducing monosaccharides naturally affords the most populous, predominantly pyranose, anomers (Jeffrey, 1990
). Previously, we have demonstrated that exceptions to this rule may be found among 1-amino-1-deoxy-ketoses. Only four acyclic ketosamine structures have been accurately characterized by X-ray diffraction so far (Mossine et al., 1995
, 2002
, 2009
); however, it was suggested that the hydrophobic nature of the amino substituents may play a supporting role in stabilization of the unique structures (Mossine et al., 2009
). Given that the concept of acyclic intermediates is essential for understanding the mechanisms of many enzymatic and non-enzymatic transformations of carbohydrates in general (see, for example, Buchholz & Seibel, 2008
; Wang et al., 2014
) and natural fructosamines in particular (Nursten, 2005
), the availability of precise structural knowledge on the open-chain 1-amino-1-deoxy-ketoses is of interest to the field. We report here on the structure of title compound, alternatively named as D-fructose-N-methyl-p-anisidine, C14H21NO6, (I)
, aiming to expand this knowledge.
2. Structural commentary
The molecular structure and atomic numbering for (I)
are shown in Fig. 1
. The molecule may be considered as a conjugate of a carbohydrate, 1-amino-1-deoxy-D-fructose, and an aromatic amine, N-methyl-p-anisidine, which are joined through the common amino group. The carbohydrate portion in (I)
exists in the acyclic keto form. Remarkably, in the aqueous solution of (I)
, the acyclic keto form is a minor constituent of the established equilibrium, at 10.3% of the total population as follows from the 13C NMR data (Table 1
). The predominant β-pyranose anomer (52.0%) and smaller proportions of the β-furanose, α-pyranose, and α-furanose cyclic forms constitute the rest of the equilibrium composition (Fig. 2
).
Compound | α-pyranose | β-pyranose | α-furanose | β-furanose | acyclic, keto | Crystalline isomers | (I) | 2.1 | 52.0 | 4.9 | 30.6 | 10.3 | acyclic keto | FruNMptia | 2.1 | 49.9 | 4.8 | 32.2 | 11.0 | acyclic keto | FruNEpcaa | 2.0 | 48.7 | 4.2 | 32.3 | 12.7 | acyclic keto | Fruptia,b | 3.5 | 61.0 | 9.4 | 24.2 | 1.9 | β-pyranose | FruAllac | 2.2 | 47.4 | 4.5 | 33.6 | 12.3 | β-pyranose | Fructosamined | 5.0 | 70.8 | 11.2 | 12.3 | 0.8 | β-pyranose | FruAibe | 3.0 | 75.6 | 10.1 | 10.4 | <0.7 | β-pyranose | Notes: (a) Mossine et al. (2009 ); (b) Gomez de Anderez et al. (1996 ); (c) Mossine et al. (2009a ); (d) Mossine et al. (2009b ); (e) Mossine et al. (2018 ). | |
| Figure 1 Atomic numbering and displacement ellipsoids at the 50% probability level for (I) . Intramolecular O—H⋯O interactions are shown as dotted lines. |
| Figure 2 Isomerization equilibrium of (I) in solution. |
The carbohydrate fragment of the molecule is in the zigzag conformation, having four out of six of its carbon atoms, C3, C4, C5, and C6, located in one plane. The conformation around the carbonyl group is also nearly flat and involves atoms N1, C1, C2, O2, C3, and O3, with the carbonyl O2 in the syn-periplanar position with respect to both N1 and O3 [respective torsion angles are 11.7 (3) and −7.0 (3)°]. This type of conformation is preferred for the β-aminocarbonyl group, due to influence of the σC—H → σC=O* and σC—H → πC=O* hyperconjugation in conditions when interaction between the nitrogen lone pair (LP) and the carbonyl π*-system is not significant (Ducati et al., 2006
). Indeed, the LP—N1—C1—C2 torsion angle estimate is close to 180° in (I)
. In the sugar portion of (I)
, the average C—O bond distances (1.43 ± 0.01Å) and the valence angles in hydroxyl groups are close to the average values for a number of crystalline alditol structures (Jeffrey & Kim, 1970
) and acyclic ketosamines (Mossine et al., 1995
, 2002
, 2009
). Two heteroatom contacts, O3—H⋯O4 and O6—H⋯O5, although weakly directional (Table 2
), are cooperatively integrated into the hydrogen-bonding scheme (see Section 3) and thus are good candidates to qualify for intramolecular hydrogen bonds. The tertiary amino group geometry is a flattened pyramid, with the distance from the N1 apex to the C1–C7–C13 base being 0.219 Å and the average base-face dihedral angle 17.2°. The N1—C7 distance, at 1.403 Å, is significantly shorter than the distances from N1 to the aliphatic carbons C1 and C13 [1.444 (3) and 1.455 (3) Å]. Such geometry is characteristic for amino groups with a mixed sp3/sp2 hybridization, likely due to a partial resonance of the nitrogen p-electrons with a neighboring π-system, such as the benzene ring in (I)
. In the solid-state 13C NMR spectrum (Fig. 3
), the peaks corresponding to the carbons C1, C7, and C13 are split, indicating a conformational dimorphism of the tertiary amino group, possibly due to an inversion of configuration at the N1 atom.
D—H⋯A | D—H | H⋯A | D⋯A | D—H⋯A | O3—H3O⋯O4 | 0.87 (3) | 2.67 (3) | 2.962 (2) | 101 (3) | O3—H3O⋯O5i | 0.87 (3) | 1.94 (3) | 2.747 (2) | 153 (2) | O4—H4O⋯O6ii | 0.80 (3) | 1.90 (3) | 2.700 (2) | 176 (3) | O5—H5O⋯O3iii | 0.86 (3) | 1.86 (3) | 2.702 (2) | 165 (3) | O6—H6O⋯O5 | 0.82 (3) | 2.56 (3) | 2.918 (2) | 108 (3) | O6—H6O⋯O4iv | 0.82 (3) | 1.95 (3) | 2.704 (2) | 154 (3) | Symmetry codes: (i) x, y+1, z; (ii) ; (iii) ; (iv) x, y-1, z. | |
| Figure 3 Solid-state 13C NMR spectrum of powdered crystalline (I) . |
3. Supramolecular features
Compound (I)
crystallizes in the monoclinic space group P21, with two equivalent molecules per unit cell. The molecular packing of (I)
features `hydrophilic' and `hydrophobic' layers propagating in the ab plane (Fig. 4
). The carbohydrate residues form a two-dimensional network of hydrogen bonds organized as a system of two homodromic infinite chains, with ⋯O3—H⋯O5—H⋯ and ⋯O4—H⋯O6—H⋯ recurrent sequences of intermolecular hydrogen bonds. These chains are topologically connected by the intramolecular short heteroatom contacts O3—H⋯O4 and O6—H⋯O5. Basic hydrogen-bonding patterns of the resulting network are depicted in Fig. 5
and include fused homodromic R88(16) and antidromic R22(4) rings (the pattern notation according to Bernstein et al., 1995
). The intermolecular heteroatom contacts that define the hydrogen bonding in (I)
are not confined exclusively to the carbohydrate portion of the molecule (Fig. 6
), however. In addition, there are two close C—H⋯O2 contacts involving the carbonyl group, and two short C—H⋯π contacts between the methyl groups and the benzene ring centroids (Cg1), which may qualify as weak hydrogen bonds (Table 3
, Fig. 6
). The Hirshfeld surface analysis (Spackman & Jayatilaka, 2009
) revealed that a major proportion of the intermolecular contacts in crystal structure of (I)
is provided by non- or low-polar interactions of the H⋯H and C⋯H type (Fig. 7
and Table 4
).
C—H⋯A | C—H | H⋯A | C⋯A | C—H⋯A | Symmetry | C1—H1A⋯O2 | 0.99 | 2.48 | 3.386 (3) | 152 | x, y − 1, z | C14—H14B⋯O2 | 0.98 | 2.52 | 3.311 (3) | 138 | -x + 1, y − , −z + 1 | C14—H14A⋯Cg1 | 0.98 | 2.95 | 3.747 (3) | 139 | -x + 1, y − , −z + 1 | C13—H13C⋯Cg1 | 0.98 | 2.80 | 3.539 (2) | 133 | -x, y + , −z + 1 | | |
Compound | Conformation | O⋯H | H⋯H | C⋯H | Other contacts | (I) | acyclic keto | 32.3 | 52.8 | 13.2 | N⋯H 1.6; C⋯C 0.1 | FruNMptia | acyclic keto | 26.5 | 59.8 | 11.8 | N⋯H 1.6; C⋯C 0.3 | FruNEpcaa | acyclic keto | 23.1 | 50.1 | 8.6 | N⋯C 0.5; C⋯C 1.3; Cl⋯H 13.1; Cl⋯C 3.4 | FruNAllab | β-pyranose | 15.2 | 67.7 | 16.9 | C⋯C 0.1 | FruNBn2c | β-pyranose | 16.5 | 64.2 | 19.2 | C⋯O 0.1 | TagNMBnd | α-pyranose | 20.6 | 65.8 | 13.5 | O⋯O 0.1 | Notes: (a) Mossine et al. (2009 ); (b) Mossine et al. (2009a ); (c) Hou et al. (2001 ); (d) Pérez et al. (1978 ). | |
| Figure 4 The molecular packing in (I) . Color code for crystallographic axes: red – a, green – b, blue – c. Hydrogen bonds are shown as cyan dotted lines. |
| Figure 5 Hydrogen-bonding pattern in the crystal structure of (I) . |
| Figure 6 Views of the Hirshfeld surface for (I) mapped over: (a) the electrostatic potential in the range −0.0966 to +0.1843 a.u. The red and blue colors represent the distribution of the negative and positive electrostatic potential, respectively; (b) the de function, in the range 0.683 to 2.484 Å, calculated for the external contact atoms in the crystal. Shown are molecular fragments involved in the C—H⋯π interactions (black dotted lines) and the shortest H⋯H contact (red dotted line). |
| Figure 7 (a) The full two-dimensional fingerprint plot for (I) and those delineated for the specific contacts: (b) O⋯H; (c) H⋯H; (d) C⋯H. |
4. Database survey
A search of SciFinder, Google Scholar, and the Cambridge Structural Database (Groom et al., 2016
) by both structure and chemical names for 1-deoxy-1-(N-methyl-p-methoxyphenylamino)-D-fructose returned no references; hence compound (I)
is new. There are four closely related structures, namely D-fructose-N-methyl-p-toluidine (FruNMpti, CCDC 717802), D-fructose-p-toluidine (Frupti, CCDC 126260), D-fructose-N-ethyl-p-chloroaniline (FruNEpca, CCDC 717803), and D-fructose-N-allylaniline (FruNAlla, CCDC 717417). Each of these 1-amino-1-deoxy-D-fructose derivatives features an aromatic substituent at the amino group. They also display a similar to (I)
distribution of the cyclic and acyclic tautomeric forms in solutions (Table 1
). However, only FruNMpti and FruNEpca were reported to adopt the acyclic keto conformations in crystalline state. Frupti (Gomez de Anderez et al., 1996
), FruNAlla (Mossine et al., 2009a
), as well as the rest of the 1-amino-1-deoxy-D-fructose derivatives whose structures were solved by X-ray diffraction methods (about 15 structures so far), crystallize in the β-D-fructopyranose anomeric form (Table 1
). The unusual propensity of some 1-amino-1-deoxy-D-fructose derivatives, including (I)
, to crystallize in a thermodynamically unfavored acyclic form is difficult to explain, given that the number of the available solved structures is thus far too small. Modelling the energies of intermolecular interactions experienced by these molecules in solutions versus crystal environments was beyond the goals of the current study. However, some initial clues can be derived from analysis of data compiled in Table 1
, this work, as well as in Table 1
from our previous study (Mossine et al., 2009
). First, only fructosamine derivatives decorated with an aromatic amino substituent can, but not always, crystallize as the acyclic keto tautomer. As pointed out in Section 2, a neighboring π-system may resonate with the amine p-electrons thus making them unavailable for σ-bonding. Next, among N-aryl derivatives of 1-amino-1-deoxy-D-fructose, only those lacking a proton bound to the tertiary amino group can crystallize in the acyclic form. Indeed, no hydrogen bonds involving N1 were detected in acyclic (I)
, FruNMpti or FruNEpca (Mossine et al., 2009
). In contrast, the structures of Frupti, FruAlla and all the rest of the 1-amino-1-deoxy-D-fructose derivatives reveal at least one hydrogen-mediated intramolecular heteroatom contact between the amino nitrogen atom and an oxygen atom originating from the carbohydrate portion of the molecule, most often the anomeric O2. Thus, the inability of the amino group to form stable intramolecular hydrogen bonds with the carbohydrate portion plays a role in stabilization of the acyclic tautomer. Finally, a comparative Hirshfeld surfaces analysis (Table 4
) of these structures suggests that the extended linear conformation of the acyclic tautomer may require more of the `hydrophilic space' available in the crystal structure, as compared to the pyranose anomers. This argument also seems to be supported by an observation that an increase in size of the N-substituents, such as from methyl or ethyl (in FruNMpti and FruNEpca) to allyl or butyl (in FruAlla and D-fructose-N-butylaniline), leads to a loss of propensity to crystallize in the acyclic form (Mossine et al., 2009
).
5. Synthesis and crystallization
The preparation of (I)
was performed following a protocol described previously (Mossine et al., 2009
). Briefly, a mixture of 5.4 g (0.03 moles) of D-glucose, 2.7 g (0.022 moles) of p-anisidine and 0.55 mL of 3-mercaptopropionic acid catalyst/antioxidant was stirred for 6 h in 12 mL of isopropanol in a screw-capped glass vial at 360 K. The reaction progress was followed by TLC. The purification step included an ion-exchange on Amberlite IRN-77 (H+), with 0.2 M NH4OH in 50% ethanol as an eluant, and was followed by flash filtration on a short silica column using 5% MeOH in CH2Cl2 as an eluant. Crystallization of the compound was aided by the addition of a small amount of acetone to the syrupy evaporation residue. The crystals were filtered off, washed with acetone and dried in vacuo over CaCl2, yield 1.6 g (27%, based on starting amine) of colorless prisms. Major (β-pyranose anomer) peaks (ppm) in the 13C NMR spectrum in D2O/pyridine: 154.14 (C10); 148.12 (C7); 117.86, 117.11 (C8, C12); 116.96, 116.80 (C9, C11); 101.56 (C2); 72.98 (C4); 71.99 (C3, C5); 65.90 (C6); 63.21 (C1); 57.84 (C14); 42.62 (C13). The 13C CPMAS–TOSS spectrum of finely powdered crystalline (I)
is shown in Fig. 3
and the minor peak assignments are listed in Supplementary Table S1.
6. Refinement
Crystal data, data collection and structure refinement details are summarized in Table 5
. Hydroxy and nitrogen-bound H atoms were located in difference-Fourier analyses and were allowed to refine fully. Other H atoms were placed at calculated positions and treated as riding, with C—H = 0.98 Å (methyl), 0.99 Å (methylene) or 1.00 Å (methine) and with Uiso(H) = 1.2Ueq(methine or methylene) or 1.5Ueq(methyl). The Flack absolute structure parameter determined [0.3 (5) for 1149 quotients (Parsons et al., 2013
)] is consistent with the (3S,4R,5R) configuration which was assigned for this chain system on the basis of the known configuration for the starting material D-glucose (McNaught, 1996
).
Crystal data | Chemical formula | C14H21NO6 | Mr | 299.32 | Crystal system, space group | Monoclinic, P21 | Temperature (K) | 100 | a, b, c (Å) | 10.8002 (15), 5.1439 (7), 13.3931 (19) | β (°) | 98.382 (1) | V (Å3) | 736.11 (18) | Z | 2 | Radiation type | Mo Kα | μ (mm−1) | 0.11 | Crystal size (mm) | 0.35 × 0.15 × 0.12 | | Data collection | Diffractometer | Bruker APEXII CCD area detector | Absorption correction | Multi-scan (SADABS; Sheldrick (2003 ) | Tmin, Tmax | 0.88, 0.99 | No. of measured, independent and observed [I > 2σ(I)] reflections | 8031, 2993, 2788 | Rint | 0.026 | (sin θ/λ)max (Å−1) | 0.625 | | Refinement | R[F2 > 2σ(F2)], wR(F2), S | 0.031, 0.082, 1.04 | No. of reflections | 2993 | No. of parameters | 208 | No. of restraints | 1 | H-atom treatment | H atoms treated by a mixture of independent and constrained refinement | Δρmax, Δρmin (e Å−3) | 0.19, −0.15 | Absolute structure | Flack x determined using 1149 quotients [(I+)−(I−)]/[(I+)+(I−)] (Parsons et al., 2013 ) | Absolute structure parameter | 0.3 (5) | Computer programs: SMART and SAINT (Bruker, 1998 ), SHELXS97 (Sheldrick, 2008 ), SHELXL2017/1 (Sheldrick, 2015 ), X-SEED (Barbour, 2001 ), Mercury (Macrae et al., 2008 ), CIFTAB (Sheldrick, 2008 ) and publCIF (Westrip, 2010 ). | |
Supporting information
Data collection: SMART (Bruker, 1998); cell refinement: SAINT (Bruker, 1998); data reduction: SAINT (Bruker, 1998); program(s) used to solve structure: SHELXS97 (Sheldrick, 2008); program(s) used to refine structure: SHELXL2017/1 (Sheldrick, 2015); molecular graphics: X-SEED (Barbour, 2001) and Mercury (Macrae et al., 2008); software used to prepare material for publication: CIFTAB (Sheldrick, 2008) and publCIF (Westrip, 2010).
1-Deoxy-1-[(4-methoxyphenyl)(methyl)amino]-
D-fructose
top Crystal data top C14H21NO6 | F(000) = 320 |
Mr = 299.32 | Dx = 1.350 Mg m−3 |
Monoclinic, P21 | Mo Kα radiation, λ = 0.71073 Å |
a = 10.8002 (15) Å | Cell parameters from 3774 reflections |
b = 5.1439 (7) Å | θ = 2.6–25.8° |
c = 13.3931 (19) Å | µ = 0.11 mm−1 |
β = 98.382 (1)° | T = 100 K |
V = 736.11 (18) Å3 | Prism, colourless |
Z = 2 | 0.35 × 0.15 × 0.12 mm |
Data collection top Bruker APEXII CCD area detector diffractometer | 2788 reflections with I > 2σ(I) |
ω scans | Rint = 0.026 |
Absorption correction: multi-scan (SADABS; Sheldrick (2003) | θmax = 26.4°, θmin = 1.9° |
Tmin = 0.88, Tmax = 0.99 | h = −13→13 |
8031 measured reflections | k = −6→6 |
2993 independent reflections | l = −16→16 |
Refinement top Refinement on F2 | Hydrogen site location: mixed |
Least-squares matrix: full | H atoms treated by a mixture of independent and constrained refinement |
R[F2 > 2σ(F2)] = 0.031 | w = 1/[σ2(Fo2) + (0.0443P)2 + 0.0803P] where P = (Fo2 + 2Fc2)/3 |
wR(F2) = 0.082 | (Δ/σ)max < 0.001 |
S = 1.04 | Δρmax = 0.19 e Å−3 |
2993 reflections | Δρmin = −0.15 e Å−3 |
208 parameters | Absolute structure: Flack x determined using 1149 quotients [(I+)-(I-)]/[(I+)+(I-)] (Parsons et al., 2013) |
1 restraint | Absolute structure parameter: 0.3 (5) |
Special details top Geometry. All esds (except the esd in the dihedral angle between two l.s. planes) are estimated using the full covariance matrix. The cell esds are taken into account individually in the estimation of esds in distances, angles and torsion angles; correlations between esds in cell parameters are only used when they are defined by crystal symmetry. An approximate (isotropic) treatment of cell esds is used for estimating esds involving l.s. planes. |
Fractional atomic coordinates and isotropic or equivalent isotropic displacement parameters (Å2) top | x | y | z | Uiso*/Ueq | |
N1 | 0.10655 (16) | 0.5973 (4) | 0.64477 (12) | 0.0275 (4) | |
C1 | 0.15040 (19) | 0.4862 (4) | 0.74257 (15) | 0.0267 (4) | |
H1A | 0.189953 | 0.316322 | 0.732685 | 0.032* | |
H1B | 0.077318 | 0.453299 | 0.777617 | 0.032* | |
O2 | 0.26415 (14) | 0.8787 (3) | 0.79127 (12) | 0.0321 (4) | |
C2 | 0.24391 (18) | 0.6543 (4) | 0.81040 (15) | 0.0240 (4) | |
O3 | 0.40317 (13) | 0.6914 (3) | 0.95778 (11) | 0.0268 (3) | |
C3 | 0.31015 (18) | 0.5253 (4) | 0.90646 (15) | 0.0229 (4) | |
H3 | 0.352434 | 0.364549 | 0.886440 | 0.027* | |
O4 | 0.13795 (14) | 0.6653 (3) | 0.99012 (12) | 0.0281 (3) | |
C4 | 0.21360 (18) | 0.4440 (4) | 0.97432 (14) | 0.0220 (4) | |
H4 | 0.159267 | 0.302656 | 0.940569 | 0.026* | |
O5 | 0.35348 (13) | 0.1285 (3) | 1.06292 (11) | 0.0270 (3) | |
C5 | 0.27762 (17) | 0.3493 (4) | 1.07730 (15) | 0.0231 (4) | |
H5 | 0.332604 | 0.491086 | 1.109907 | 0.028* | |
O6 | 0.09673 (13) | 0.0803 (3) | 1.10474 (11) | 0.0285 (3) | |
C6 | 0.18494 (19) | 0.2727 (5) | 1.14715 (15) | 0.0285 (5) | |
H6A | 0.231641 | 0.204885 | 1.210924 | 0.034* | |
H6B | 0.138815 | 0.429609 | 1.163615 | 0.034* | |
C7 | 0.18576 (18) | 0.5977 (4) | 0.57050 (15) | 0.0250 (4) | |
C8 | 0.2825 (2) | 0.4181 (5) | 0.57062 (16) | 0.0322 (5) | |
H8 | 0.300894 | 0.301939 | 0.625936 | 0.039* | |
C9 | 0.3529 (2) | 0.4053 (5) | 0.49151 (17) | 0.0352 (5) | |
H9 | 0.417860 | 0.280262 | 0.493123 | 0.042* | |
O10 | 0.39239 (16) | 0.5762 (4) | 0.32846 (12) | 0.0437 (4) | |
C10 | 0.3286 (2) | 0.5736 (5) | 0.41076 (16) | 0.0319 (5) | |
C11 | 0.2348 (2) | 0.7560 (5) | 0.41043 (17) | 0.0352 (5) | |
H11 | 0.218288 | 0.874400 | 0.355652 | 0.042* | |
C12 | 0.1644 (2) | 0.7690 (5) | 0.48871 (16) | 0.0316 (5) | |
H12 | 0.100474 | 0.896471 | 0.486890 | 0.038* | |
C13 | 0.0158 (2) | 0.8065 (5) | 0.64424 (17) | 0.0330 (5) | |
H13A | 0.059076 | 0.974339 | 0.646201 | 0.050* | |
H13B | −0.027328 | 0.790523 | 0.703438 | 0.050* | |
H13C | −0.045390 | 0.795655 | 0.582714 | 0.050* | |
C14 | 0.4858 (2) | 0.3812 (7) | 0.3254 (2) | 0.0474 (7) | |
H14A | 0.551236 | 0.401318 | 0.383834 | 0.071* | |
H14B | 0.522812 | 0.399739 | 0.263176 | 0.071* | |
H14C | 0.447653 | 0.208769 | 0.327161 | 0.071* | |
H5O | 0.430 (3) | 0.175 (7) | 1.061 (2) | 0.050 (8)* | |
H4O | 0.069 (3) | 0.643 (7) | 0.959 (2) | 0.052 (9)* | |
H6O | 0.133 (3) | −0.034 (6) | 1.078 (2) | 0.047 (8)* | |
H3O | 0.368 (2) | 0.835 (6) | 0.974 (2) | 0.041 (8)* | |
Atomic displacement parameters (Å2) top | U11 | U22 | U33 | U12 | U13 | U23 |
N1 | 0.0270 (9) | 0.0286 (9) | 0.0267 (9) | 0.0036 (8) | 0.0032 (7) | 0.0003 (7) |
C1 | 0.0277 (11) | 0.0254 (10) | 0.0268 (10) | −0.0020 (9) | 0.0032 (8) | 0.0011 (8) |
O2 | 0.0344 (8) | 0.0240 (8) | 0.0372 (9) | −0.0023 (7) | 0.0026 (7) | 0.0027 (7) |
C2 | 0.0207 (9) | 0.0242 (10) | 0.0288 (10) | 0.0003 (8) | 0.0099 (8) | −0.0007 (8) |
O3 | 0.0166 (7) | 0.0272 (8) | 0.0365 (8) | −0.0027 (6) | 0.0038 (6) | −0.0025 (6) |
C3 | 0.0180 (9) | 0.0223 (10) | 0.0287 (10) | −0.0003 (7) | 0.0048 (8) | −0.0013 (8) |
O4 | 0.0178 (7) | 0.0281 (8) | 0.0383 (8) | 0.0029 (6) | 0.0040 (6) | −0.0057 (6) |
C4 | 0.0172 (9) | 0.0220 (10) | 0.0274 (10) | 0.0011 (7) | 0.0052 (8) | −0.0023 (8) |
O5 | 0.0168 (7) | 0.0276 (8) | 0.0368 (8) | 0.0007 (6) | 0.0045 (6) | 0.0031 (6) |
C5 | 0.0164 (9) | 0.0270 (10) | 0.0261 (10) | −0.0009 (8) | 0.0037 (8) | −0.0033 (8) |
O6 | 0.0190 (7) | 0.0306 (8) | 0.0370 (8) | −0.0016 (6) | 0.0081 (6) | −0.0033 (7) |
C6 | 0.0229 (10) | 0.0363 (12) | 0.0266 (10) | −0.0059 (9) | 0.0043 (8) | −0.0020 (10) |
C7 | 0.0244 (9) | 0.0235 (10) | 0.0258 (10) | −0.0023 (8) | −0.0012 (8) | −0.0044 (8) |
C8 | 0.0327 (11) | 0.0344 (12) | 0.0288 (11) | 0.0070 (10) | 0.0021 (9) | 0.0038 (9) |
C9 | 0.0313 (11) | 0.0390 (14) | 0.0353 (12) | 0.0084 (10) | 0.0054 (9) | −0.0012 (10) |
O10 | 0.0399 (9) | 0.0585 (11) | 0.0354 (9) | 0.0012 (9) | 0.0143 (7) | −0.0009 (8) |
C10 | 0.0281 (11) | 0.0398 (13) | 0.0283 (10) | −0.0043 (10) | 0.0055 (8) | −0.0046 (10) |
C11 | 0.0376 (13) | 0.0344 (12) | 0.0335 (12) | 0.0000 (10) | 0.0054 (10) | 0.0080 (10) |
C12 | 0.0326 (11) | 0.0263 (11) | 0.0355 (12) | 0.0058 (9) | 0.0033 (9) | 0.0040 (10) |
C13 | 0.0294 (11) | 0.0350 (13) | 0.0346 (11) | 0.0058 (10) | 0.0044 (9) | −0.0031 (10) |
C14 | 0.0331 (12) | 0.0692 (19) | 0.0416 (14) | 0.0003 (13) | 0.0115 (10) | −0.0126 (13) |
Geometric parameters (Å, º) top N1—C7 | 1.403 (3) | O6—H6O | 0.82 (3) |
N1—C1 | 1.444 (3) | C6—H6A | 0.9900 |
N1—C13 | 1.455 (3) | C6—H6B | 0.9900 |
C1—C2 | 1.525 (3) | C7—C8 | 1.395 (3) |
C1—H1A | 0.9900 | C7—C12 | 1.399 (3) |
C1—H1B | 0.9900 | C8—C9 | 1.392 (3) |
O2—C2 | 1.209 (3) | C8—H8 | 0.9500 |
C2—C3 | 1.529 (3) | C9—C10 | 1.380 (3) |
O3—C3 | 1.418 (2) | C9—H9 | 0.9500 |
O3—H3O | 0.87 (3) | O10—C10 | 1.382 (3) |
C3—C4 | 1.538 (3) | O10—C14 | 1.428 (3) |
C3—H3 | 1.0000 | C10—C11 | 1.381 (3) |
O4—C4 | 1.435 (2) | C11—C12 | 1.383 (3) |
O4—H4O | 0.80 (3) | C11—H11 | 0.9500 |
C4—C5 | 1.530 (3) | C12—H12 | 0.9500 |
C4—H4 | 1.0000 | C13—H13A | 0.9800 |
O5—C5 | 1.430 (2) | C13—H13B | 0.9800 |
O5—H5O | 0.86 (3) | C13—H13C | 0.9800 |
C5—C6 | 1.518 (3) | C14—H14A | 0.9800 |
C5—H5 | 1.0000 | C14—H14B | 0.9800 |
O6—C6 | 1.432 (3) | C14—H14C | 0.9800 |
| | | |
C7—N1—C1 | 119.35 (16) | C5—C6—H6A | 108.9 |
C7—N1—C13 | 118.38 (17) | O6—C6—H6B | 108.9 |
C1—N1—C13 | 115.40 (17) | C5—C6—H6B | 108.9 |
N1—C1—C2 | 114.64 (18) | H6A—C6—H6B | 107.7 |
N1—C1—H1A | 108.6 | C8—C7—C12 | 117.14 (18) |
C2—C1—H1A | 108.6 | C8—C7—N1 | 122.18 (19) |
N1—C1—H1B | 108.6 | C12—C7—N1 | 120.53 (19) |
C2—C1—H1B | 108.6 | C9—C8—C7 | 121.5 (2) |
H1A—C1—H1B | 107.6 | C9—C8—H8 | 119.3 |
O2—C2—C1 | 122.7 (2) | C7—C8—H8 | 119.3 |
O2—C2—C3 | 121.04 (19) | C10—C9—C8 | 120.3 (2) |
C1—C2—C3 | 116.29 (17) | C10—C9—H9 | 119.9 |
C3—O3—H3O | 109.2 (18) | C8—C9—H9 | 119.9 |
O3—C3—C2 | 110.97 (16) | C10—O10—C14 | 116.9 (2) |
O3—C3—C4 | 111.77 (16) | C9—C10—C11 | 118.96 (19) |
C2—C3—C4 | 109.93 (15) | C9—C10—O10 | 124.8 (2) |
O3—C3—H3 | 108.0 | C11—C10—O10 | 116.2 (2) |
C2—C3—H3 | 108.0 | C10—C11—C12 | 121.0 (2) |
C4—C3—H3 | 108.0 | C10—C11—H11 | 119.5 |
C4—O4—H4O | 108 (2) | C12—C11—H11 | 119.5 |
O4—C4—C5 | 108.19 (15) | C11—C12—C7 | 121.1 (2) |
O4—C4—C3 | 108.74 (16) | C11—C12—H12 | 119.4 |
C5—C4—C3 | 111.30 (15) | C7—C12—H12 | 119.4 |
O4—C4—H4 | 109.5 | N1—C13—H13A | 109.5 |
C5—C4—H4 | 109.5 | N1—C13—H13B | 109.5 |
C3—C4—H4 | 109.5 | H13A—C13—H13B | 109.5 |
C5—O5—H5O | 111 (2) | N1—C13—H13C | 109.5 |
O5—C5—C6 | 108.62 (17) | H13A—C13—H13C | 109.5 |
O5—C5—C4 | 108.92 (15) | H13B—C13—H13C | 109.5 |
C6—C5—C4 | 112.72 (16) | O10—C14—H14A | 109.5 |
O5—C5—H5 | 108.8 | O10—C14—H14B | 109.5 |
C6—C5—H5 | 108.8 | H14A—C14—H14B | 109.5 |
C4—C5—H5 | 108.8 | O10—C14—H14C | 109.5 |
C6—O6—H6O | 110 (2) | H14A—C14—H14C | 109.5 |
O6—C6—C5 | 113.26 (17) | H14B—C14—H14C | 109.5 |
O6—C6—H6A | 108.9 | | |
| | | |
C7—N1—C1—C2 | 75.2 (2) | C4—C5—C6—O6 | 55.7 (2) |
C13—N1—C1—C2 | −75.3 (2) | C1—N1—C7—C8 | 24.6 (3) |
N1—C1—C2—O2 | 11.7 (3) | C13—N1—C7—C8 | 174.25 (19) |
N1—C1—C2—C3 | −169.53 (16) | C1—N1—C7—C12 | −160.0 (2) |
O2—C2—C3—O3 | −7.0 (3) | C13—N1—C7—C12 | −10.3 (3) |
C1—C2—C3—O3 | 174.20 (16) | C12—C7—C8—C9 | −1.6 (3) |
O2—C2—C3—C4 | 117.2 (2) | N1—C7—C8—C9 | 174.0 (2) |
C1—C2—C3—C4 | −61.6 (2) | C7—C8—C9—C10 | 0.5 (4) |
O3—C3—C4—O4 | 70.7 (2) | C8—C9—C10—C11 | 0.8 (4) |
C2—C3—C4—O4 | −53.0 (2) | C8—C9—C10—O10 | −179.7 (2) |
O3—C3—C4—C5 | −48.3 (2) | C14—O10—C10—C9 | 3.2 (3) |
C2—C3—C4—C5 | −172.04 (16) | C14—O10—C10—C11 | −177.2 (2) |
O4—C4—C5—O5 | 180.00 (15) | C9—C10—C11—C12 | −1.0 (4) |
C3—C4—C5—O5 | −60.6 (2) | O10—C10—C11—C12 | 179.5 (2) |
O4—C4—C5—C6 | 59.4 (2) | C10—C11—C12—C7 | −0.1 (4) |
C3—C4—C5—C6 | 178.77 (18) | C8—C7—C12—C11 | 1.4 (3) |
O5—C5—C6—O6 | −65.1 (2) | N1—C7—C12—C11 | −174.2 (2) |
Hydrogen-bond geometry (Å, º) top D—H···A | D—H | H···A | D···A | D—H···A |
O3—H3O···O4 | 0.87 (3) | 2.67 (3) | 2.962 (2) | 101 (3) |
O3—H3O···O5i | 0.87 (3) | 1.94 (3) | 2.747 (2) | 153 (2) |
O4—H4O···O6ii | 0.80 (3) | 1.90 (3) | 2.700 (2) | 176 (3) |
O5—H5O···O3iii | 0.86 (3) | 1.86 (3) | 2.702 (2) | 165 (3) |
O6—H6O···O5 | 0.82 (3) | 2.56 (3) | 2.918 (2) | 108 (3) |
O6—H6O···O4iv | 0.82 (3) | 1.95 (3) | 2.704 (2) | 154 (3) |
Symmetry codes: (i) x, y+1, z; (ii) −x, y+1/2, −z+2; (iii) −x+1, y−1/2, −z+2; (iv) x, y−1, z. |
Distribution (%) of cyclic and acyclic forms of some 1-amino-1-deoxy-D-fructose derivatives in D2O/pyridine (1:1) at 293 K, as estimated from the 13C NMR spectra, and in the crystalline state topCompound | α-pyranose | β-pyranose | α-furanose | β-furanose | acyclic, keto | Crystalline isomers |
(I) | 2.1 | 52.0 | 4.9 | 30.6 | 10.3 | acyclic keto |
FruNMptia | 2.1 | 49.9 | 4.8 | 32.2 | 11.0 | acyclic keto |
FruNEpcaa | 2.0 | 48.7 | 4.2 | 32.3 | 12.7 | acyclic keto |
Fruptia,b | 3.5 | 61.0 | 9.4 | 24.2 | 1.9 | β-pyranose |
FruAllac | 2.2 | 47.4 | 4.5 | 33.6 | 12.3 | β-pyranose |
Fructosamined | 5.0 | 70.8 | 11.2 | 12.3 | 0.8 | β-pyranose |
FruAibe | 3.0 | 75.6 | 10.1 | 10.4 | <0.7 | β-pyranose |
Notes: (a) Mossine et al. (2009); (b) Gomez de Anderez et al. (1996); (c) Mossine et al. (2009a); (d) Mossine et al. (2009b); (e) Mossine et al. (2018). |
Suspected C—H···A contacts (Å, °) topC—H···A | C—H | H···A | C···A | C—H···A | Symmetry |
C1—H1A···O2 | 0.99 | 2.48 | 3.386 (3) | 152 | x, y - 1, z |
C14—H14B···O2 | 0.98 | 2.52 | 3.311 (3) | 138 | -x + 1, y - 1/2, -z + 1 |
C14—H14A···Cg1 | 0.98 | 2.95 | 3.747 (3) | 139 | -x + 1, y - 1/2, -z + 1 |
C13—H13C···Cg1 | 0.98 | 2.80 | 3.539 (2) | 133 | -x, y + 1/2, -z + 1 |
Contributions (%) of specific contact types to the Hirshfeld surfaces of 1-amino-1-deoxy-D-fructose derivatives topCompound | Conformation | O···H | H···H | C···H | Other contacts |
(I) | acyclic keto | 32.3 | 52.8 | 13.2 | N···H 1.6; C···C 0.1 |
FruNMptia | acyclic keto | 26.5 | 59.8 | 11.8 | N···H 1.6; C···C 0.3 |
FruNEpcaa | acyclic keto | 23.1 | 50.1 | 8.6 | N···C 0.5; C···C 1.3; Cl···H 13.1; Cl···C 3.4 |
FruNAllab | β-pyranose | 15.2 | 67.7 | 16.9 | C···C 0.1 |
FruNBn2c | β-pyranose | 16.5 | 64.2 | 19.2 | C···O 0.1 |
TagNMBnd | α-pyranose | 20.6 | 65.8 | 13.5 | O···O 0.1 |
Notes: (a) Mossine et al. (2009); (b) Mossine et al. (2009a); (c) Hou et al. (2001); (d) Pérez et al. (1978). |
Supplementary Table S1. Chemical shifts (ppm) of peaks for selected carbon atoms in a 13C NMR spectrum of (I) in D2O/pyridine (1:1) at 293 K and in the solid state topCarbon | α-pyranose | β-pyranose | α-furanose | β-furanose | acyclic keto | solid state |
C1 | 60.97 | 63.21 | 61.28 | 61.28 | 62.41 | 57.23 |
C2 | 101.13 | 101.56 | 108.26 | 105.62 | 215.08 | 210.04 |
C3 | 73.62 | 71.99 | 84.62 | 79.73 | 78.93 | 77.80 |
C4 | 74.92 | 72.98 | 80.26 | 77.42 | 74.96 | 69.59 |
C5 | 66.66 | 71.99 | 85.64 | 83.77 | 73.45 | 69.59 |
C6 | 63.7 | 65.90 | 64.37 | 65.14 | 65.81 | 64.70 |
C13 | n.r. | 42.62 | n.r. | 42.09 | 41.85 | 39.56 |
Funding information
Funding for this research was provided by: University of Missouri Agriculture Experiment Station Chemical Laboratories.
References
Angyal, S. J. (1992). Adv. Carbohydr. Chem. Biochem. 49, 19–35. CrossRef CAS Web of Science Google Scholar
Barbour, L. J. (2001). J. Supramol. Chem. 1, 189–191. CrossRef CAS Google Scholar
Bernstein, J., Davis, R. E., Shimoni, L. & Chang, N.-L. (1995). Angew. Chem. Int. Ed. Engl. 34, 1555–1573. CrossRef CAS Web of Science Google Scholar
Bruker. (1998). SMART and SAINT-Plus. Bruker AXS Inc., Madison, Wisconsin, USA. Google Scholar
Buchholz, K. & Seibel, J. (2008). Carbohydr. Res. 343, 1966–1979. Web of Science CrossRef PubMed CAS Google Scholar
Ducati, L. C., Rittner, R. & Custódio, R. (2006). J. Mol. Struct. Theochem, 766, 177–183. Web of Science CrossRef CAS Google Scholar
Gomez de Anderez, D., Gil, H., Helliwell, M. & Mata Segreda, J. (1996). Acta Cryst. C52, 252–254. CSD CrossRef CAS IUCr Journals Google Scholar
Groom, C. R., Bruno, I. J., Lightfoot, M. P. & Ward, S. C. (2016). Acta Cryst. B72, 171–179. Web of Science CSD CrossRef IUCr Journals Google Scholar
Hou, Y., Wu, X., Xie, W., Braunschweiger, P. G. & Wang, P. G. (2001). Tetrahedron Lett. 42, 825–829. Web of Science CSD CrossRef CAS Google Scholar
Jeffrey, G. A. (1990). Acta Cryst. B46, 89–103. CrossRef CAS Web of Science IUCr Journals Google Scholar
Jeffrey, G. A. & Kim, H. S. (1970). Carbohydr. Res. 14, 207–216. CrossRef CAS Web of Science Google Scholar
Macrae, C. F., Bruno, I. J., Chisholm, J. A., Edgington, P. R., McCabe, P., Pidcock, E., Rodriguez-Monge, L., Taylor, R., van de Streek, J. & Wood, P. A. (2008). J. Appl. Cryst. 41, 466–470. Web of Science CSD CrossRef CAS IUCr Journals Google Scholar
McNaught, A. D. (1996). Pure Appl. Chem. 68, 1919–2008. CrossRef CAS Web of Science Google Scholar
Mossine, V. V., Barnes, C. L., Chance, D. L. & Mawhinney, T. P. (2009). Angew. Chem. Int. Ed. 48, 5517–5520. Web of Science CSD CrossRef CAS Google Scholar
Mossine, V. V., Barnes, C. L., Feather, M. S. & Mawhinney, T. P. (2002). J. Am. Chem. Soc. 124, 15178–15179. Web of Science CSD CrossRef PubMed CAS Google Scholar
Mossine, V. V., Barnes, C. L., Glinsky, G. V. & Feather, M. S. (1995). Carbohydr. Lett. 1, 355–362. CAS Google Scholar
Mossine, V. V., Barnes, C. L. & Mawhinney, T. P. (2009a). Carbohydr. Res. 344, 948–951. Web of Science CSD CrossRef PubMed CAS Google Scholar
Mossine, V. V., Barnes, C. L. & Mawhinney, T. P. (2009b). J. Carbohydr. Chem. 28, 245–263. Web of Science CSD CrossRef CAS Google Scholar
Mossine, V. V., Barnes, C. L. & Mawhinney, T. P. (2018). Acta Cryst. E74, 72–77. Web of Science CSD CrossRef IUCr Journals Google Scholar
Nursten, H. (2005). The Maillard Reaction: Chemistry, Biochemistry and Implications. Cambridge: The Royal Society of Chemistry. Google Scholar
Parsons, S., Flack, H. D. & Wagner, T. (2013). Acta Cryst. B69, 249–259. Web of Science CrossRef CAS IUCr Journals Google Scholar
Pérez, S., López-Castro, A. & Márquez, R. (1978). Acta Cryst. B34, 2341–2344. CSD CrossRef IUCr Journals Web of Science Google Scholar
Sheldrick, G. M. (2003). SADABS. University of Göttingen, Germany. Google Scholar
Sheldrick, G. M. (2008). Acta Cryst. A64, 112–122. Web of Science CrossRef CAS IUCr Journals Google Scholar
Sheldrick, G. M. (2015). Acta Cryst. C71, 3–8. Web of Science CrossRef IUCr Journals Google Scholar
Spackman, M. A. & Jayatilaka, D. (2009). CrystEngComm, 11, 19–32. Web of Science CrossRef CAS Google Scholar
Wang, T., Nolte, M. W. & Shanks, B. H. (2014). Green Chem. 16, 548–572. Web of Science CrossRef CAS Google Scholar
Westrip, S. P. (2010). J. Appl. Cryst. 43, 920–925. Web of Science CrossRef CAS IUCr Journals Google Scholar
This is an open-access article distributed under the terms of the Creative Commons Attribution (CC-BY) Licence, which permits unrestricted use, distribution, and reproduction in any medium, provided the original authors and source are cited.
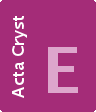 | CRYSTALLOGRAPHIC COMMUNICATIONS |
ISSN: 2056-9890
Open

access