1. Chemical context
While formerly the purview of all-alkyl substituents (Hogarth, 2005
; Heard, 2005
), recent work in the chemistry of dithiocarbamate ligands, −S2CN(R)R′, has increasingly seen the inclusion of oxygen atoms in these N-bound groups (Hogarth et al., 2009
), leading to different chemistry/biochemistry. Oxygen can be present as a hydroxyl group, giving rise to supramolecular aggregation patterns based on hydrogen bonding for otherwise non-aggregating species (Tan et al., 2016
; Jotani et al., 2017
) or as an ether, giving rise to compounds with biological activity (Ferreira et al., 2012
). Organotin dithiocarbamates have long been known to possess biological activity, in particular as anti-tumour and anti-bacterial agents (Tiekink, 2008
). In keeping with the aforementioned, several recent studies have appeared investigating the biological activity of metal dithiocarbamates where the ligand contains at least one 2-methoxyethyl substituent (Khan et al., 2013
, 2016
), including anti-bacterial potential of organotins (Mohamad, Awang, Kamaludin & Abu Bakar, 2016
; Mohamad, Awang & Kamaludin, 2016
). The latter studies have been augmented by several structural investigations in recent times (Mohamad, Awang, Jotani & Tiekink, 2016
; Mohamad, Awang, Kamaludin, Jotani et al., 2016
; Mohamad et al., 2017
). In a continuation of these structural studies, herein, the crystal and molecular structures of (n-Bu)2Sn[S2CN(CH2CH2OCH3)2]2 (I)
and (C6H5)3Sn[S2CN(CH3)CH2CH2OCH3] (II)
are reported along with a Hirshfeld surface analysis to provide more details on the molecular packing, which generally lacks directional intermolecular interactions.
1.1. Structural commentary
The tin atom in (I)
, Fig. 1
a, lies on a crystallographic twofold axis so that the asymmetric unit comprises half a molecule. The dithiocarbamate ligand coordinates to the tin atom with quite disparate Sn—S bond lengths with Δ(Sn—S) = d(Sn—Slong) − (Sn—S)short = 0.38 Å, Table 1
. The disparity in the Sn—S bond lengths is reflected in systematic differences in the C—S bonds lengths with the bond associated with the stronger Sn—S1 bond being significantly longer, i.e. by about 0.03 Å, than the C—S bond associated with the weaker Sn—S2 bond. The coordination environment is completed by two α-carbon atoms of the n-butyl substituents. The resultant C2S4 donor set defines a skew-trapezoidal bipyramidal geometry with the tin-bound organic substituents lying over the weaker Sn—S2 bonds, which subtend an angle at the tin atom approximately 50° wider than that subtended by the S1 atoms, Table 1
. The 2-methoxyethyl groups lie to either side of the S2CN residue and have very similar conformations, as seen in the values of the C1—N1—C2—C3, N1—C2—C3—O1 and C2—C3—O1—C4 torsion angles of −94.1 (4), −67.4 (4) and −177.1 (3)°, indicating that − anti-clinal, − syn-clinal and − anti-periplanar descriptors, respectively, are in effect. For the O2-methoxyethyl group, the equivalent torsion angles are −82.0 (4), −70.3 (4) and −169.1 (3)°. The independent n-butyl substituent has an all-trans (+ anti-periplanar) conformation, as seen in the values of the Sn—C8—C9—C10 and C8—C9—C10— C11 torsion angles of 172.9 (2) and 176.3 (3)°, respectively.
Sn—S1 | 2.5503 (9) | S1—C1 | 1.736 (3) | Sn—S2 | 2.9300 (9) | S2—C1 | 1.702 (3) | Sn—C8 | 2.131 (3) | | | | | | | S1—Sn—S2 | 65.13 (3) | S2—Sn—C8 | 83.95 (9) | S1—Sn—S1i | 87.95 (4) | S1—Sn—C8i | 104.64 (9) | S2—Sn—S2i | 141.79 (3) | S2—Sn—C8i | 82.96 (9) | S1—Sn—C8 | 104.38 (9) | C8—Sn—C8i | 139.25 (17) | Symmetry code: (i) . | |
| Figure 1 The molecular structures of (a) (I) and (b) (II) , showing the atom-labelling schemes and displacement ellipsoids at the 50% probability level. Unlabelled atoms in (a) are related by the symmetry operation x, y, − z. |
The molecule in (II)
, Fig. 1
b, lies on a general position and has a quite distinct coordination geometry owing to the presence of three tin-bound phenyl groups. As for (I)
, the dithiocarbamate ligand coordinates in an asymmetric mode with Δ(Sn—S) being 0.55 Å. Consistent with the greater disparity in Sn—S bond lengths, the difference in the associated C—S bond lengths in (II)
is greater cf. (I)
, i.e. nearly 0.07 Å, Table 2
. The increased asymmetry in the mode of coordination of the dithiocarbamate ligand in (II)
, cf. (I)
, is correlated with the reduced Lewis acidity of the tin atom in the triorganotin compound, (II)
, compared with that in the diorganotin compound, (I)
. The angles subtended at the tin atom vary from a narrow 64.37 (2)° for the S1—Sn—S2 chelate angle to 155.54 (8)° for S2—Sn—C11. The C3S2 donor set approximates a trigonal–bipyramidal geometry with the value of τ, an indicator of a five-coordinate coordination geometry, being 0.61, cf. 1.0 for an ideal trigonal bipyramid and 0.0 for an ideal square pyramid (Addison et al., 1984
). If the coordination geometry is considered as being based on a C3S donor set, the range of tetrahedral angles is 91.17 (8)°, for S1—Sn—C11, to 119.09 (7)°, for S1—Sn—C31. The C21—Sn—C31 angle, at 115.55 (10)°, is wider by 10° than the other C—Sn—C angles, a result correlated with the close approach of the S2 atom. The 2-methoxyethyl group has a very similar conformation to the O1-methoxyethyl group in (I)
, with the values of the C1—N1—C3—C4, N1—C3—C4—O1 and C5—O1—C4—C3 torsion angles being 95.1 (3), 81.8 (3) and 178.7 (3)°, respectively.
Sn—S1 | 2.4711 (7) | Sn—C11 | 2.162 (3) | Sn—S2 | 3.0180 (7) | Sn—C21 | 2.136 (3) | S1—C1 | 1.755 (3) | Sn—C31 | 2.133 (2) | S2—C1 | 1.686 (3) | | | | | | | S1—Sn—S2 | 64.37 (2) | S2—Sn—C21 | 87.38 (7) | S1—Sn—C11 | 91.17 (8) | S2—Sn—C31 | 87.83 (7) | S1—Sn—C21 | 115.84 (7) | C11—Sn—C21 | 104.11 (10) | S1—Sn—C31 | 119.09 (7) | C11—Sn—C31 | 105.78 (10) | S2—Sn—C11 | 155.54 (8) | C21—Sn—C31 | 115.55 (10) | | |
3. Hirshfeld surface analysis
The Hirshfeld surface calculations for the organotin derivatives (I)
and (II)
were performed in accord with recent work on related organotin dithiocarbamate compounds (Mohamad et al., 2017
), and these exhibit different intermolecular environments as described below.
The bright-red spots near each of the methoxy-O1 and -O2, and methylene-H4A and H6B atoms lying on both the sides of twofold symmetry axis on the Hirshfeld surfaces mapped over dnorm for (I)
in Fig. 4
a and b represent the dominant intermolecular C—H⋯O contacts, Table 3
. In addition, the bright-red spots appearing near the methoxy-H8B and butyl-H8A atoms in Fig. 4
c indicate the significant influence of intra-layer H⋯H contacts, Table 5
. On the Hirshfeld surface mapped over the electrostatic potential for (I)
shown in Fig. 5
a and b, the donors and acceptors are represented with blue and red regions around the respective atoms corresponding to positive and negative potentials, respectively.
Contact | Distance | Symmetry operation | (I) | | | H4B⋯H8A | 2.00 | −x, − y, 1 − z | H5A⋯H6B | 2.21 | − x, − y, 1 − z | H8B⋯H10B | 2.37 | −x, 1 − y, 1 − z | H10B⋯H10B | 2.37 | −x, 1 − y, 1 − z | (II) | | | H14⋯H33 | 2.37 | 1 + x, 1 + y, z | H16⋯H33 | 2.25 | x, 1 + y, z | H22⋯H34 | 2.33 | x, 1 + y, z | C1⋯H3B | 2.86 | −x, − y, 2 − z | C14⋯H4A | 2.85 | 1 − x, 1 − y, 2 − z | | |
| Figure 4 Views of Hirshfeld surface for (I) mapped over dnorm in the range −0.163 to +1.302, highlighting (a) and (b) intermolecular methylene-C—H⋯O(methoxy) interactions and (c) short intra-layer H⋯H contacts between methoxy- and butyl-hydrogen atoms H4B and H8A as sky-blue dashed lines. |
| Figure 5 Views of Hirshfeld surface mapped over the electrostatic potential (the red and blue regions represent negative and positive electrostatic potentials, respectively) for: (a) and (b) a molecule of (I) in the range −0.054 to +0.036 au and (c) a molecule of (II) in the range ±0.036 au. |
The Hirshfeld surfaces mapped over dnorm for (II)
(not shown), indicate the absence of significant directional interactions operating in the crystal as no characteristic red spots appear on the surface. The blue and red regions on the Hirshfeld surface mapped over electrostatic potential for (II)
in Fig. 5
c are due to polarization of charges near the respective functional groups and do not represent any significant interaction in the crystal. The weak intermolecular C—H⋯π contact and intra-layer interatomic H⋯H contacts (Table 5
) present in the crystal of (II)
are illustrated in Fig. 6
.
| Figure 6 Views of the Hirshfeld surface for (II) mapped with the shape-index property showing (a) intermolecular C—H⋯π/π⋯H—C contacts and (b) short interatomic H⋯H contacts through black-dashed lines. |
The overall two-dimensional fingerprint plots for (I)
and (II)
, Fig. 7
a and b, reveal the distinct supramolecular associations in their crystals. The terminal methoxy-ethyl and coordinated n-butyl substituents in (I)
form significant intra-layer H⋯H contacts in comparison to (II)
, Table 5
. This fact is also indicated in the fingerprint plots delineated into H⋯H contacts (McKinnon et al., 2007
), showing a short thick spike at de + di ∼ 2.0 Å and the distribution of points with greater density in (de, di) range ∼1.0 to 1.2 Å for (I)
in Fig. 7
a, and a small peak at de + di ∼ 2.2 Å with relatively few points at de + di < 2.4 Å for (II)
in Fig. 7
b. The fingerprint plot delineated into O⋯H/H⋯O contacts for (I)
, Fig. 7
a, characterizes intermolecular C—H⋯O interactions as the pair of forceps-like tips at de + di ∼ 2.5 Å. A low percentage contribution due to O⋯H/H⋯O contacts is noted for (II)
, as summarized in Table 6
. The relatively high, i.e. 29.1%, contribution from C⋯H/H⋯C contacts to the Hirshfeld surfaces of (II)
is due to the presence of tin-bound phenyl substituents and the resulting short interatomic C⋯H/H⋯C contacts, Table 5
, and intermolecular C—H⋯π contact, Table 4
, viewed as the pair of peaks at de + di ∼ 2.8 Å and the distribution of points around de + di ∼ 2.9 Å in both the wings of its delineated fingerprint plot, Fig. 7b
. Although S⋯H/H⋯S contacts have significant contributions to the Hirshfeld surfaces of (I)
and (II)
, as summarized in Table 6
, their interatomic distances are farther than sum of their van der Waals radii, i.e. de + di > 3.0 Å, Fig. 7
, and hence do not have a structure-directing influence on the molecular packing. The small contributions from other contacts in (I)
and (II)
also have negligible impact in the respective crystals.
Contact | % contribution in (I) | % contribution in (II) | H⋯H | 77.9 | 58.9 | S⋯H/H⋯S | 12.2 | 7.3 | C⋯H/H⋯C | 1.6 | 29.1 | O⋯H/H⋯O | 7.9 | 2.5 | N⋯H/H⋯N | 0.4 | 0.7 | C⋯S/S⋯C | 0.0 | 1.3 | S⋯O/O⋯S | 0.0 | 0.1 | Sn⋯H/N⋯Sn | 0.0 | 0.1 | | |
| Figure 7 Comparison of the full two-dimensional fingerprint plots for (I) and (II) , and the plots delineated into (a) H⋯H, O⋯H/H⋯O and S⋯H/H⋯S contacts and (b) H⋯H, C⋯H/H⋯C and S⋯H/H⋯S contacts. |
4. Database survey
It is well documented that organotin dithiocarbamates, R′′xSn(S2CNRR′)4–x, can adopt a variety of coordination geometries, especially for x = 2 (Tiekink, 2008
). The structural motifs for the x = 2 series were recently summarized (Zaldi et al., 2017
) and four structural motifs recognized. With a trapezoidal bipyramidal geometry being observed in (I)
, this structure conforms to the common motif for the x = 2 structures. There is one other diorganotin structure with the same dithiocarbamate ligand, viz. the R′′ = C6H5 compound (Mohamad, Awang, Jotani et al., 2016
). This, too, adopts the common trapezoidal bipyramidal geometry although a good number of other derivatives with R′′ = Ph adopt octahedral geometries, such as in (C6H5)2Sn[S2CN(CH3)CH2CH2OCH3]2 (Muthalib et al., 2014
) featuring the same dithiocarbamate ligand as in (II)
. The observed anisobidentate mode of coordination for the dithiocarbamate ligand in (II)
is as expected and in fact is the norm for x = 3 structures which may be described as having 4 + 1 coordination geometries (Tiekink, 2008
).
5. Synthesis and crystallization
All chemicals and solvents were used as purchased without purification. The melting points were determined using an automated melting point apparatus (MPA 120 EZ-Melt). Carbon, hydrogen, nitrogen and sulfur analyses were performed on a Leco CHNS-932 Elemental Analyzer. The IR spectra were obtained on a Perkin Elmer Spectrum GX from 4000 to 400 cm−1. NMR spectra were recorded at room temperature on Bruker AVANCE 400 lll HD in CDCl3.
Synthesis of (I)
: bis(2-methoxyethyl)amine (Aldrich; 1.48 ml, 10 mmol) dissolved in ethanol (30 ml) was stirred for 30 min. Then, carbon disulfide (0.6 ml, 10 mmol) in cold ethanol was added and the resulting mixture was stirred for 2 h. A 25% ammonia solution (1–2 ml) was added to generate basic conditions. Then, di-n-butyltin(IV) dichloride (Aldrich; 1.52 g, 5 mmol) dissolved in ethanol (20–30 ml) was added dropwise into the solution and stirring was continued for 2 h. All reactions were carried out at 277 K. The precipitate that formed was dried and collected. Colourless prisms were harvested from the slow evaporation of its chloroform:ethanol (2:1 v/v) solution. Yield: 72%. M.p. 341–342 K. Elemental analysis: calculated (%): C 40.68, H 7.14, N 4.31, S 19.75. Found (%): C 41.76, H 6.07, N 4.91, S 19.25. IR (KBr cm−1): 1487 ν(C—N), 992 ν(C—S), 544 ν(Sn—C), 386 ν(Sn—S). 1H NMR (CDCl3): δ 4.13 (2H, O—CH2); 3.70 (2H, N—CH2); 3.35 (3H, O—CH3); 1.45–2.05 (6H, Sn—CH2—CH2—CH2–), 0.94 (3H, CH2—CH3). 13C NMR (CDCl3): δ 201.52 (NCS2); 70.07 (O—CH2); 55.59 (N—CH2); 59.01 (O—CH3); 34.26 Sn—CH2; 28.55 Sn—CH2CH2; 26.41 Sn—CH2CH2CH2; 13.87 CH2CH3. 119Sn NMR (CDCl3): δ −335.5.
Synthesis of (II)
: The synthesis of (II)
was carried out in the same manner as for (I)
using (2-methoxyethyl)methylamine (Santa Cruz Biotechnology; 1.1 ml, 10 mmol) and triphenyltin(IV) chloride (Merck; 3.85 g, 10 mmol). Crystallization in the form of colourless slabs was from its chloroform:ethanol (1:2 v/v) solution. Yield: 78%. M.p. 366-367 K. Elemental analysis: calculated (%): C 53.71, H 4.89, N 2.72, S 12.47. Found (%): C 54.28, H 5.26, N 2.73, S 12.5. IR (KBr cm−1): 1477 ν(C—N), 997 ν(C—S), 527 ν(Sn—C), 451 ν(Sn—S). 1H NMR (CDCl3): δ 7.41–7.82 (15H, Sn—C6H5); 4.05 (2H, O—CH2); 3.71 (2H, N—CH2); 3.51 (3H, O—CH3); 3.38 (3H, N—CH3). 13C NMR (CDCl3): δ 196.97 (NCS2); 128.25–142.28 (C-aromatic); 70.09 (O—CH2); 59.06 (N—CH2); 58.10 (O—CH3); 45.81 (N—CH3);. 119Sn NMR (CDCl3): δ −183.8.
6. Refinement
Crystal data, data collection and structure refinement details are summarized in Table 7
. Carbon-bound H atoms were placed in calculated positions (C—H = 0.95–0.99 Å) and were included in the refinement in the riding-model approximation, with Uiso(H) set to 1.2–1.5Ueq(C). For (I)
, the maximum and minimum residual electron density peaks of 2.18 and 0.88 e Å−3, respectively, were located 0.88 and 1.03 Å from the S1 and Sn atoms, respectively. For (II)
, the maximum and minimum residual electron density peaks of 2.21 and 1.82 e Å−3, respectively, were located 0.96 and 0.76 Å from the Sn atom.
| (I) | (II) | Crystal data | Chemical formula | [Sn(C4H9)2(C7H14NO2S2)2] | [Sn(C6H5)3(C5H10NOS2)] | Mr | 649.54 | 514.25 | Crystal system, space group | Monoclinic, C2/c | Triclinic, P![[\overline{1}]](teximages/hb7731fi1.gif) | Temperature (K) | 173 | 148 | a, b, c (Å) | 25.8819 (17), 7.1272 (4), 16.4146 (11) | 7.6258 (3), 10.2178 (3), 14.8621 (6) | α, β, γ (°) | 90, 97.282 (6), 90 | 91.976 (3), 90.655 (3), 107.875 (3) | V (Å3) | 3003.5 (3) | 1101.19 (7) | Z | 4 | 2 | Radiation type | Mo Kα | Mo Kα | μ (mm−1) | 1.16 | 1.36 | Crystal size (mm) | 0.30 × 0.15 × 0.05 | 0.50 × 0.30 × 0.30 | | Data collection | Diffractometer | Agilent Technologies SuperNova Dual diffractometer with Atlas detector | Agilent Technologies SuperNova Dual diffractometer with Atlas detector | Absorption correction | Multi-scan (CrysAlis PRO; Agilent, 2015 ) | Multi-scan (CrysAlis PRO; Agilent, 2015 ) | Tmin, Tmax | 0.247, 1.000 | 0.479, 1.000 | No. of measured, independent and observed [I > 2σ(I)] reflections | 18349, 4631, 3678 | 11916, 6547, 6089 | Rint | 0.054 | 0.046 | (sin θ/λ)max (Å−1) | 0.738 | 0.739 | | Refinement | R[F2 > 2σ(F2)], wR(F2), S | 0.048, 0.128, 1.05 | 0.040, 0.114, 1.09 | No. of reflections | 4631 | 6547 | No. of parameters | 153 | 255 | H-atom treatment | H-atom parameters constrained | H-atom parameters constrained | Δρmax, Δρmin (e Å−3) | 2.18, −0.88 | 2.21, −1.82 | Computer programs: CrysAlis PRO (Agilent, 2015 ), SHELXS97 (Sheldrick, 2008 ), SHELXL2014 (Sheldrick, 2015 ), ORTEP-3 for Windows (Farrugia, 2012 ), DIAMOND (Brandenburg, 2006 ) and publCIF (Westrip, 2010 ). | |
Supporting information
For both structures, data collection: CrysAlis PRO (Agilent, 2015); cell refinement: CrysAlis PRO (Agilent, 2015); data reduction: CrysAlis PRO (Agilent, 2015); program(s) used to solve structure: SHELXS97 (Sheldrick, 2008); program(s) used to refine structure: SHELXL2014 (Sheldrick, 2015). Molecular graphics: ORTEP-3 for Windows (Farrugia, 2012) and DIAMOND (Brandenburg, 2006) for (I); ORTEP-3 for Windows (Farrugia, 2012) for (II). For both structures, software used to prepare material for publication: publCIF (Westrip, 2010).
Bis[
N,
N-bis(2-methoxyethyl)dithiocarbamato-
κ2S,
S']di-
n-butyltin(IV) (I)
top Crystal data top [Sn(C4H9)2(C7H14NO2S2)2] | F(000) = 1352 |
Mr = 649.54 | Dx = 1.436 Mg m−3 |
Monoclinic, C2/c | Mo Kα radiation, λ = 0.71073 Å |
a = 25.8819 (17) Å | Cell parameters from 5103 reflections |
b = 7.1272 (4) Å | θ = 3.9–30.2° |
c = 16.4146 (11) Å | µ = 1.16 mm−1 |
β = 97.282 (6)° | T = 173 K |
V = 3003.5 (3) Å3 | Prism, colourless |
Z = 4 | 0.30 × 0.15 × 0.05 mm |
Data collection top Agilent Technologies SuperNova Dual diffractometer with Atlas detector | 4631 independent reflections |
Radiation source: SuperNova (Mo) X-ray Source | 3678 reflections with I > 2σ(I) |
Mirror monochromator | Rint = 0.054 |
Detector resolution: 10.4041 pixels mm-1 | θmax = 31.6°, θmin = 3.3° |
ω scan | h = −36→37 |
Absorption correction: multi-scan (CrysAlis Pro; Agilent, 2015) | k = −9→9 |
Tmin = 0.247, Tmax = 1.000 | l = −18→23 |
18349 measured reflections | |
Refinement top Refinement on F2 | 0 restraints |
Least-squares matrix: full | Hydrogen site location: inferred from neighbouring sites |
R[F2 > 2σ(F2)] = 0.048 | H-atom parameters constrained |
wR(F2) = 0.128 | w = 1/[σ2(Fo2) + (0.0581P)2 + 4.9909P] where P = (Fo2 + 2Fc2)/3 |
S = 1.05 | (Δ/σ)max < 0.001 |
4631 reflections | Δρmax = 2.18 e Å−3 |
153 parameters | Δρmin = −0.88 e Å−3 |
Special details top Geometry. All esds (except the esd in the dihedral angle between two l.s. planes) are estimated using the full covariance matrix. The cell esds are taken into account individually in the estimation of esds in distances, angles and torsion angles; correlations between esds in cell parameters are only used when they are defined by crystal symmetry. An approximate (isotropic) treatment of cell esds is used for estimating esds involving l.s. planes. |
Refinement. The maximum and minimum residual electron density peaks of 2.18 and 0.88 eÅ-3, respectively, were located 0.88 Å and 1.03 Å from the S1 and Sn atoms, respectively. |
Fractional atomic coordinates and isotropic or equivalent isotropic displacement parameters (Å2) top | x | y | z | Uiso*/Ueq | |
Sn | 0.0000 | 0.22626 (4) | 0.2500 | 0.03003 (11) | |
S1 | 0.06302 (3) | −0.03124 (11) | 0.30644 (5) | 0.03538 (19) | |
S2 | 0.09910 (3) | 0.36081 (12) | 0.33633 (5) | 0.03628 (19) | |
O1 | 0.18723 (10) | −0.1322 (4) | 0.54586 (15) | 0.0420 (6) | |
O2 | 0.26265 (10) | 0.1284 (4) | 0.34048 (17) | 0.0468 (6) | |
N1 | 0.15552 (10) | 0.0612 (4) | 0.38750 (16) | 0.0307 (5) | |
C1 | 0.11053 (12) | 0.1264 (4) | 0.34726 (18) | 0.0300 (6) | |
C2 | 0.16660 (13) | −0.1410 (5) | 0.4000 (2) | 0.0348 (7) | |
H2A | 0.2041 | −0.1635 | 0.3970 | 0.042* | |
H2B | 0.1465 | −0.2127 | 0.3550 | 0.042* | |
C3 | 0.15321 (15) | −0.2133 (5) | 0.4811 (2) | 0.0378 (7) | |
H3A | 0.1167 | −0.1807 | 0.4872 | 0.045* | |
H3B | 0.1566 | −0.3516 | 0.4830 | 0.045* | |
C4 | 0.17861 (15) | −0.2007 (6) | 0.6240 (2) | 0.0444 (9) | |
H4A | 0.1816 | −0.3378 | 0.6247 | 0.067* | |
H4B | 0.1437 | −0.1645 | 0.6351 | 0.067* | |
H4C | 0.2046 | −0.1473 | 0.6662 | 0.067* | |
C5 | 0.19574 (13) | 0.1927 (5) | 0.42487 (19) | 0.0329 (7) | |
H5A | 0.2181 | 0.1273 | 0.4695 | 0.039* | |
H5B | 0.1785 | 0.2983 | 0.4498 | 0.039* | |
C6 | 0.22976 (13) | 0.2705 (5) | 0.3646 (2) | 0.0342 (7) | |
H6A | 0.2076 | 0.3201 | 0.3157 | 0.041* | |
H6B | 0.2511 | 0.3751 | 0.3903 | 0.041* | |
C7 | 0.30157 (16) | 0.2029 (6) | 0.2963 (3) | 0.0475 (9) | |
H7A | 0.3247 | 0.2850 | 0.3323 | 0.071* | |
H7B | 0.2851 | 0.2752 | 0.2492 | 0.071* | |
H7C | 0.3219 | 0.1000 | 0.2767 | 0.071* | |
C8 | −0.03139 (13) | 0.3304 (5) | 0.35511 (19) | 0.0311 (6) | |
H8A | −0.0673 | 0.2822 | 0.3543 | 0.037* | |
H8B | −0.0104 | 0.2810 | 0.4051 | 0.037* | |
C9 | −0.03254 (13) | 0.5440 (4) | 0.36019 (19) | 0.0323 (6) | |
H9A | 0.0036 | 0.5920 | 0.3684 | 0.039* | |
H9B | −0.0498 | 0.5949 | 0.3075 | 0.039* | |
C10 | −0.06123 (13) | 0.6138 (5) | 0.42990 (19) | 0.0341 (7) | |
H10A | −0.0980 | 0.5726 | 0.4197 | 0.041* | |
H10B | −0.0454 | 0.5562 | 0.4821 | 0.041* | |
C11 | −0.05949 (17) | 0.8260 (5) | 0.4386 (2) | 0.0454 (9) | |
H11A | −0.0752 | 0.8838 | 0.3872 | 0.068* | |
H11B | −0.0232 | 0.8671 | 0.4510 | 0.068* | |
H11C | −0.0790 | 0.8639 | 0.4834 | 0.068* | |
Atomic displacement parameters (Å2) top | U11 | U22 | U33 | U12 | U13 | U23 |
Sn | 0.03662 (18) | 0.02227 (17) | 0.03257 (17) | 0.000 | 0.00965 (12) | 0.000 |
S1 | 0.0400 (4) | 0.0212 (4) | 0.0445 (4) | −0.0060 (3) | 0.0036 (3) | −0.0035 (3) |
S2 | 0.0399 (4) | 0.0252 (4) | 0.0433 (4) | −0.0045 (3) | 0.0037 (3) | 0.0006 (3) |
O1 | 0.0456 (14) | 0.0362 (15) | 0.0443 (13) | −0.0097 (11) | 0.0063 (11) | 0.0091 (11) |
O2 | 0.0485 (15) | 0.0295 (14) | 0.0657 (16) | −0.0004 (11) | 0.0197 (12) | 0.0066 (12) |
N1 | 0.0393 (14) | 0.0201 (13) | 0.0338 (13) | −0.0038 (10) | 0.0094 (11) | −0.0006 (10) |
C1 | 0.0391 (16) | 0.0268 (16) | 0.0267 (13) | −0.0013 (12) | 0.0148 (12) | 0.0008 (11) |
C2 | 0.0406 (17) | 0.0218 (16) | 0.0433 (17) | 0.0027 (12) | 0.0102 (14) | −0.0027 (13) |
C3 | 0.0461 (19) | 0.0186 (16) | 0.0496 (19) | −0.0032 (12) | 0.0101 (15) | 0.0015 (13) |
C4 | 0.044 (2) | 0.037 (2) | 0.052 (2) | −0.0035 (15) | 0.0053 (16) | 0.0186 (16) |
C5 | 0.0356 (16) | 0.0309 (18) | 0.0314 (15) | −0.0100 (12) | 0.0011 (12) | 0.0012 (12) |
C6 | 0.0376 (17) | 0.0263 (17) | 0.0384 (17) | −0.0085 (12) | 0.0038 (13) | 0.0054 (12) |
C7 | 0.044 (2) | 0.043 (2) | 0.058 (2) | −0.0004 (16) | 0.0157 (17) | 0.0078 (18) |
C8 | 0.0376 (16) | 0.0239 (15) | 0.0337 (15) | −0.0023 (12) | 0.0117 (12) | 0.0002 (12) |
C9 | 0.0403 (17) | 0.0241 (16) | 0.0338 (15) | −0.0014 (12) | 0.0093 (13) | −0.0002 (12) |
C10 | 0.0401 (17) | 0.0281 (17) | 0.0355 (15) | 0.0019 (13) | 0.0104 (13) | −0.0005 (12) |
C11 | 0.067 (2) | 0.0281 (19) | 0.0429 (19) | 0.0084 (16) | 0.0138 (17) | −0.0051 (15) |
Geometric parameters (Å, º) top Sn—S1 | 2.5503 (9) | C4—H4C | 0.9800 |
Sn—S1i | 2.5504 (9) | C5—C6 | 1.511 (4) |
Sn—S2 | 2.9300 (9) | C5—H5A | 0.9900 |
Sn—S2i | 2.9300 (9) | C5—H5B | 0.9900 |
Sn—C8 | 2.131 (3) | C6—H6A | 0.9900 |
Sn—C8i | 2.131 (3) | C6—H6B | 0.9900 |
S1—C1 | 1.736 (3) | C7—H7A | 0.9800 |
S2—C1 | 1.702 (3) | C7—H7B | 0.9800 |
O1—C3 | 1.415 (4) | C7—H7C | 0.9800 |
O1—C4 | 1.416 (4) | C8—C9 | 1.526 (4) |
O2—C6 | 1.412 (4) | C8—H8A | 0.9900 |
O2—C7 | 1.417 (4) | C8—H8B | 0.9900 |
N1—C1 | 1.347 (4) | C9—C10 | 1.524 (4) |
N1—C5 | 1.475 (4) | C9—H9A | 0.9900 |
N1—C2 | 1.479 (4) | C9—H9B | 0.9900 |
C2—C3 | 1.508 (5) | C10—C11 | 1.519 (5) |
C2—H2A | 0.9900 | C10—H10A | 0.9900 |
C2—H2B | 0.9900 | C10—H10B | 0.9900 |
C3—H3A | 0.9900 | C11—H11A | 0.9800 |
C3—H3B | 0.9900 | C11—H11B | 0.9800 |
C4—H4A | 0.9800 | C11—H11C | 0.9800 |
C4—H4B | 0.9800 | | |
| | | |
S1—Sn—S2 | 65.13 (3) | C6—C5—H5A | 108.9 |
S1—Sn—S1i | 87.95 (4) | N1—C5—H5B | 108.9 |
S2—Sn—S2i | 141.79 (3) | C6—C5—H5B | 108.9 |
S1—Sn—C8 | 104.38 (9) | H5A—C5—H5B | 107.7 |
S2—Sn—C8 | 83.95 (9) | O2—C6—C5 | 110.0 (3) |
S1—Sn—C8i | 104.64 (9) | O2—C6—H6A | 109.7 |
C8—Sn—S1i | 104.64 (9) | C5—C6—H6A | 109.7 |
C8i—Sn—S1i | 104.38 (9) | O2—C6—H6B | 109.7 |
S2—Sn—C8i | 82.96 (9) | C5—C6—H6B | 109.7 |
S1—Sn—S2i | 153.08 (3) | H6A—C6—H6B | 108.2 |
C8—Sn—C8i | 139.25 (17) | O2—C7—H7A | 109.5 |
C1—S1—Sn | 93.64 (11) | O2—C7—H7B | 109.5 |
C1—S2—Sn | 81.93 (11) | H7A—C7—H7B | 109.5 |
C3—O1—C4 | 112.6 (3) | O2—C7—H7C | 109.5 |
C6—O2—C7 | 111.6 (3) | H7A—C7—H7C | 109.5 |
C1—N1—C5 | 120.4 (3) | H7B—C7—H7C | 109.5 |
C1—N1—C2 | 123.0 (3) | C9—C8—Sn | 113.8 (2) |
C5—N1—C2 | 116.6 (3) | C9—C8—H8A | 108.8 |
N1—C1—S2 | 121.2 (2) | Sn—C8—H8A | 108.8 |
N1—C1—S1 | 119.5 (2) | C9—C8—H8B | 108.8 |
S2—C1—S1 | 119.31 (19) | Sn—C8—H8B | 108.8 |
N1—C2—C3 | 113.2 (3) | H8A—C8—H8B | 107.7 |
N1—C2—H2A | 108.9 | C10—C9—C8 | 112.4 (3) |
C3—C2—H2A | 108.9 | C10—C9—H9A | 109.1 |
N1—C2—H2B | 108.9 | C8—C9—H9A | 109.1 |
C3—C2—H2B | 108.9 | C10—C9—H9B | 109.1 |
H2A—C2—H2B | 107.8 | C8—C9—H9B | 109.1 |
O1—C3—C2 | 109.4 (3) | H9A—C9—H9B | 107.9 |
O1—C3—H3A | 109.8 | C11—C10—C9 | 112.6 (3) |
C2—C3—H3A | 109.8 | C11—C10—H10A | 109.1 |
O1—C3—H3B | 109.8 | C9—C10—H10A | 109.1 |
C2—C3—H3B | 109.8 | C11—C10—H10B | 109.1 |
H3A—C3—H3B | 108.2 | C9—C10—H10B | 109.1 |
O1—C4—H4A | 109.5 | H10A—C10—H10B | 107.8 |
O1—C4—H4B | 109.5 | C10—C11—H11A | 109.5 |
H4A—C4—H4B | 109.5 | C10—C11—H11B | 109.5 |
O1—C4—H4C | 109.5 | H11A—C11—H11B | 109.5 |
H4A—C4—H4C | 109.5 | C10—C11—H11C | 109.5 |
H4B—C4—H4C | 109.5 | H11A—C11—H11C | 109.5 |
N1—C5—C6 | 113.6 (3) | H11B—C11—H11C | 109.5 |
N1—C5—H5A | 108.9 | | |
| | | |
C5—N1—C1—S2 | 1.4 (4) | C5—N1—C2—C3 | 83.2 (3) |
C2—N1—C1—S2 | 178.6 (2) | C4—O1—C3—C2 | −177.1 (3) |
C5—N1—C1—S1 | −178.1 (2) | N1—C2—C3—O1 | −67.4 (4) |
C2—N1—C1—S1 | −0.8 (4) | C1—N1—C5—C6 | −82.0 (4) |
Sn—S2—C1—N1 | −179.6 (2) | C2—N1—C5—C6 | 100.6 (3) |
Sn—S2—C1—S1 | −0.11 (15) | C7—O2—C6—C5 | −169.1 (3) |
Sn—S1—C1—N1 | 179.6 (2) | N1—C5—C6—O2 | −70.3 (4) |
Sn—S1—C1—S2 | 0.12 (17) | Sn—C8—C9—C10 | 172.9 (2) |
C1—N1—C2—C3 | −94.1 (4) | C8—C9—C10—C11 | 176.3 (3) |
Symmetry code: (i) −x, y, −z+1/2. |
Hydrogen-bond geometry (Å, º) topCg1 is the centroid of the N4/C5–C9 ring. |
D—H···A | D—H | H···A | D···A | D—H···A |
C4—H4A···O2ii | 0.98 | 2.55 | 3.423 (5) | 149 |
C6—H6B···O1iii | 0.99 | 2.57 | 3.553 (4) | 175 |
Symmetry codes: (ii) −x+1/2, −y−1/2, −z+1; (iii) −x+1/2, −y+1/2, −z+1. |
[
N-(2-Methoxyethyl)-
N-methyldithiocarbamato-
κ2S,
S']triphenyltin(IV) (II)
top Crystal data top [Sn(C6H5)3(C5H10NOS2)] | Z = 2 |
Mr = 514.25 | F(000) = 520 |
Triclinic, P1 | Dx = 1.551 Mg m−3 |
a = 7.6258 (3) Å | Mo Kα radiation, λ = 0.71073 Å |
b = 10.2178 (3) Å | Cell parameters from 8110 reflections |
c = 14.8621 (6) Å | θ = 4.1–31.4° |
α = 91.976 (3)° | µ = 1.36 mm−1 |
β = 90.655 (3)° | T = 148 K |
γ = 107.875 (3)° | Slab, colourless |
V = 1101.19 (7) Å3 | 0.50 × 0.30 × 0.30 mm |
Data collection top Agilent Technologies SuperNova Dual diffractometer with Atlas detector | 6547 independent reflections |
Radiation source: SuperNova (Mo) X-ray Source | 6089 reflections with I > 2σ(I) |
Mirror monochromator | Rint = 0.046 |
Detector resolution: 10.4041 pixels mm-1 | θmax = 31.7°, θmin = 3.5° |
ω scan | h = −11→11 |
Absorption correction: multi-scan (CrysAlis Pro; Agilent, 2015) | k = −15→14 |
Tmin = 0.479, Tmax = 1.000 | l = −20→20 |
11916 measured reflections | |
Refinement top Refinement on F2 | 0 restraints |
Least-squares matrix: full | Hydrogen site location: inferred from neighbouring sites |
R[F2 > 2σ(F2)] = 0.040 | H-atom parameters constrained |
wR(F2) = 0.114 | w = 1/[σ2(Fo2) + (0.0671P)2 + 0.2783P] where P = (Fo2 + 2Fc2)/3 |
S = 1.09 | (Δ/σ)max = 0.001 |
6547 reflections | Δρmax = 2.21 e Å−3 |
255 parameters | Δρmin = −1.82 e Å−3 |
Special details top Geometry. All esds (except the esd in the dihedral angle between two l.s. planes) are estimated using the full covariance matrix. The cell esds are taken into account individually in the estimation of esds in distances, angles and torsion angles; correlations between esds in cell parameters are only used when they are defined by crystal symmetry. An approximate (isotropic) treatment of cell esds is used for estimating esds involving l.s. planes. |
Refinement. The maximum and minimum residual electron density peaks of 2.21 and 1.82 eÅ-3, respectively, were located 0.96 Å and 0.76 Å from the Sn atom. |
Fractional atomic coordinates and isotropic or equivalent isotropic displacement parameters (Å2) top | x | y | z | Uiso*/Ueq | |
Sn | 0.33155 (2) | 0.17167 (2) | 0.71926 (2) | 0.01646 (7) | |
S1 | 0.22626 (10) | 0.21030 (8) | 0.87168 (5) | 0.02514 (15) | |
S2 | −0.04590 (10) | −0.01260 (7) | 0.76544 (5) | 0.02473 (15) | |
O1 | −0.3089 (3) | 0.2686 (2) | 1.02520 (19) | 0.0379 (6) | |
N1 | −0.1129 (3) | 0.0832 (3) | 0.92564 (17) | 0.0254 (5) | |
C1 | 0.0058 (4) | 0.0900 (3) | 0.85949 (19) | 0.0211 (5) | |
C2 | −0.3028 (4) | −0.0073 (4) | 0.9179 (3) | 0.0358 (7) | |
H2A | −0.3842 | 0.0464 | 0.9015 | 0.054* | |
H2B | −0.3399 | −0.0496 | 0.9757 | 0.054* | |
H2C | −0.3118 | −0.0794 | 0.8713 | 0.054* | |
C3 | −0.0635 (4) | 0.1730 (4) | 1.0074 (2) | 0.0302 (6) | |
H3A | 0.0717 | 0.2002 | 1.0174 | 0.036* | |
H3B | −0.1218 | 0.1203 | 1.0596 | 0.036* | |
C4 | −0.1216 (4) | 0.3017 (3) | 1.0033 (2) | 0.0293 (6) | |
H4A | −0.0459 | 0.3734 | 1.0464 | 0.035* | |
H4B | −0.1032 | 0.3380 | 0.9421 | 0.035* | |
C5 | −0.3705 (6) | 0.3865 (4) | 1.0244 (3) | 0.0456 (9) | |
H5A | −0.2985 | 0.4559 | 1.0689 | 0.068* | |
H5B | −0.5011 | 0.3603 | 1.0395 | 0.068* | |
H5C | −0.3542 | 0.4246 | 0.9644 | 0.068* | |
C11 | 0.5881 (4) | 0.3348 (3) | 0.74340 (19) | 0.0210 (5) | |
C12 | 0.7602 (4) | 0.3153 (3) | 0.7336 (2) | 0.0249 (6) | |
H12 | 0.7669 | 0.2264 | 0.7169 | 0.030* | |
C13 | 0.9229 (4) | 0.4240 (4) | 0.7479 (2) | 0.0321 (7) | |
H13 | 1.0389 | 0.4091 | 0.7404 | 0.039* | |
C14 | 0.9145 (4) | 0.5536 (3) | 0.7729 (2) | 0.0308 (6) | |
H14 | 1.0250 | 0.6279 | 0.7821 | 0.037* | |
C15 | 0.7455 (4) | 0.5753 (3) | 0.7846 (2) | 0.0299 (6) | |
H15 | 0.7397 | 0.6641 | 0.8021 | 0.036* | |
C16 | 0.5846 (4) | 0.4662 (3) | 0.7704 (2) | 0.0262 (6) | |
H16 | 0.4691 | 0.4814 | 0.7793 | 0.031* | |
C21 | 0.2016 (4) | 0.2414 (3) | 0.61084 (18) | 0.0188 (5) | |
C22 | 0.2874 (4) | 0.3708 (3) | 0.5777 (2) | 0.0262 (6) | |
H22 | 0.3998 | 0.4270 | 0.6050 | 0.031* | |
C23 | 0.2114 (5) | 0.4192 (4) | 0.5050 (2) | 0.0345 (7) | |
H23 | 0.2704 | 0.5083 | 0.4838 | 0.041* | |
C24 | 0.0504 (5) | 0.3373 (4) | 0.4643 (2) | 0.0370 (7) | |
H24 | −0.0018 | 0.3700 | 0.4148 | 0.044* | |
C25 | −0.0359 (5) | 0.2075 (4) | 0.4951 (2) | 0.0344 (7) | |
H25 | −0.1463 | 0.1509 | 0.4663 | 0.041* | |
C26 | 0.0387 (4) | 0.1600 (3) | 0.5682 (2) | 0.0263 (6) | |
H26 | −0.0219 | 0.0712 | 0.5894 | 0.032* | |
C31 | 0.4062 (3) | −0.0098 (3) | 0.69017 (18) | 0.0175 (5) | |
C32 | 0.3456 (4) | −0.1288 (3) | 0.7390 (2) | 0.0272 (6) | |
H32 | 0.2659 | −0.1323 | 0.7881 | 0.033* | |
C33 | 0.4018 (5) | −0.2424 (3) | 0.7156 (3) | 0.0360 (8) | |
H33 | 0.3608 | −0.3231 | 0.7493 | 0.043* | |
C34 | 0.5168 (4) | −0.2389 (3) | 0.6439 (3) | 0.0312 (7) | |
H34 | 0.5535 | −0.3173 | 0.6281 | 0.037* | |
C35 | 0.5782 (4) | −0.1216 (3) | 0.5952 (2) | 0.0255 (6) | |
H35 | 0.6573 | −0.1190 | 0.5460 | 0.031* | |
C36 | 0.5237 (4) | −0.0067 (3) | 0.61873 (19) | 0.0212 (5) | |
H36 | 0.5673 | 0.0745 | 0.5857 | 0.025* | |
Atomic displacement parameters (Å2) top | U11 | U22 | U33 | U12 | U13 | U23 |
Sn | 0.01712 (11) | 0.01286 (10) | 0.01959 (11) | 0.00482 (7) | 0.00283 (7) | 0.00054 (7) |
S1 | 0.0219 (3) | 0.0277 (3) | 0.0224 (3) | 0.0028 (3) | 0.0036 (2) | −0.0031 (3) |
S2 | 0.0239 (3) | 0.0231 (3) | 0.0250 (3) | 0.0041 (3) | 0.0038 (3) | 0.0000 (3) |
O1 | 0.0331 (12) | 0.0285 (11) | 0.0553 (16) | 0.0134 (10) | 0.0124 (11) | 0.0057 (11) |
N1 | 0.0239 (12) | 0.0295 (12) | 0.0249 (12) | 0.0108 (10) | 0.0072 (9) | 0.0043 (10) |
C1 | 0.0216 (12) | 0.0201 (12) | 0.0233 (13) | 0.0085 (10) | 0.0035 (10) | 0.0037 (10) |
C2 | 0.0258 (15) | 0.0372 (17) | 0.0429 (19) | 0.0065 (13) | 0.0144 (13) | 0.0060 (14) |
C3 | 0.0348 (16) | 0.0395 (17) | 0.0213 (13) | 0.0183 (14) | 0.0079 (11) | 0.0027 (12) |
C4 | 0.0316 (15) | 0.0277 (14) | 0.0259 (14) | 0.0051 (12) | 0.0076 (12) | 0.0003 (11) |
C5 | 0.047 (2) | 0.0374 (19) | 0.060 (3) | 0.0249 (17) | −0.0004 (18) | −0.0011 (18) |
C11 | 0.0195 (12) | 0.0174 (11) | 0.0248 (13) | 0.0038 (10) | 0.0036 (10) | 0.0029 (9) |
C12 | 0.0225 (13) | 0.0212 (13) | 0.0316 (15) | 0.0079 (11) | 0.0026 (11) | −0.0024 (11) |
C13 | 0.0185 (13) | 0.0373 (17) | 0.0381 (17) | 0.0052 (12) | 0.0043 (12) | −0.0009 (13) |
C14 | 0.0248 (14) | 0.0289 (15) | 0.0293 (15) | −0.0053 (12) | 0.0018 (12) | −0.0011 (12) |
C15 | 0.0336 (16) | 0.0176 (12) | 0.0348 (16) | 0.0025 (11) | 0.0007 (12) | −0.0020 (11) |
C16 | 0.0243 (14) | 0.0192 (13) | 0.0344 (16) | 0.0059 (11) | 0.0022 (11) | −0.0018 (11) |
C21 | 0.0201 (12) | 0.0178 (11) | 0.0201 (12) | 0.0077 (10) | 0.0060 (9) | 0.0021 (9) |
C22 | 0.0267 (14) | 0.0212 (13) | 0.0312 (15) | 0.0075 (11) | 0.0026 (11) | 0.0054 (11) |
C23 | 0.0418 (18) | 0.0308 (16) | 0.0348 (17) | 0.0155 (14) | 0.0069 (14) | 0.0137 (13) |
C24 | 0.0381 (18) | 0.050 (2) | 0.0303 (16) | 0.0225 (16) | 0.0043 (13) | 0.0139 (15) |
C25 | 0.0301 (16) | 0.0466 (19) | 0.0252 (15) | 0.0096 (14) | −0.0025 (12) | 0.0034 (13) |
C26 | 0.0234 (13) | 0.0285 (14) | 0.0242 (14) | 0.0035 (11) | 0.0018 (11) | 0.0027 (11) |
C31 | 0.0161 (11) | 0.0140 (10) | 0.0219 (12) | 0.0039 (9) | 0.0019 (9) | 0.0016 (9) |
C32 | 0.0269 (14) | 0.0191 (13) | 0.0389 (16) | 0.0103 (11) | 0.0141 (12) | 0.0109 (11) |
C33 | 0.0344 (17) | 0.0191 (13) | 0.059 (2) | 0.0131 (12) | 0.0178 (15) | 0.0148 (14) |
C34 | 0.0271 (14) | 0.0196 (13) | 0.0492 (19) | 0.0111 (11) | 0.0036 (13) | −0.0033 (13) |
C35 | 0.0233 (13) | 0.0269 (14) | 0.0271 (14) | 0.0094 (11) | 0.0042 (11) | −0.0049 (11) |
C36 | 0.0212 (12) | 0.0199 (12) | 0.0220 (12) | 0.0054 (10) | 0.0036 (10) | 0.0006 (10) |
Geometric parameters (Å, º) top Sn—S1 | 2.4711 (7) | C13—H13 | 0.9500 |
Sn—S2 | 3.0180 (7) | C14—C15 | 1.385 (5) |
S1—C1 | 1.755 (3) | C14—H14 | 0.9500 |
S2—C1 | 1.686 (3) | C15—C16 | 1.390 (4) |
Sn—C11 | 2.162 (3) | C15—H15 | 0.9500 |
Sn—C21 | 2.136 (3) | C16—H16 | 0.9500 |
Sn—C31 | 2.133 (2) | C21—C22 | 1.393 (4) |
O1—C4 | 1.408 (4) | C21—C26 | 1.396 (4) |
O1—C5 | 1.420 (4) | C22—C23 | 1.395 (4) |
N1—C1 | 1.333 (4) | C22—H22 | 0.9500 |
N1—C2 | 1.460 (4) | C23—C24 | 1.375 (5) |
N1—C3 | 1.470 (4) | C23—H23 | 0.9500 |
C2—H2A | 0.9800 | C24—C25 | 1.384 (5) |
C2—H2B | 0.9800 | C24—H24 | 0.9500 |
C2—H2C | 0.9800 | C25—C26 | 1.389 (4) |
C3—C4 | 1.514 (4) | C25—H25 | 0.9500 |
C3—H3A | 0.9900 | C26—H26 | 0.9500 |
C3—H3B | 0.9900 | C31—C36 | 1.392 (4) |
C4—H4A | 0.9900 | C31—C32 | 1.393 (4) |
C4—H4B | 0.9900 | C32—C33 | 1.389 (4) |
C5—H5A | 0.9800 | C32—H32 | 0.9500 |
C5—H5B | 0.9800 | C33—C34 | 1.383 (5) |
C5—H5C | 0.9800 | C33—H33 | 0.9500 |
C11—C12 | 1.395 (4) | C34—C35 | 1.379 (5) |
C11—C16 | 1.396 (4) | C34—H34 | 0.9500 |
C12—C13 | 1.396 (4) | C35—C36 | 1.395 (4) |
C12—H12 | 0.9500 | C35—H35 | 0.9500 |
C13—C14 | 1.383 (5) | C36—H36 | 0.9500 |
| | | |
S1—Sn—S2 | 64.37 (2) | C14—C13—C12 | 119.8 (3) |
S1—Sn—C11 | 91.17 (8) | C14—C13—H13 | 120.1 |
S1—Sn—C21 | 115.84 (7) | C12—C13—H13 | 120.1 |
S1—Sn—C31 | 119.09 (7) | C13—C14—C15 | 120.2 (3) |
S2—Sn—C11 | 155.54 (8) | C13—C14—H14 | 119.9 |
S2—Sn—C21 | 87.38 (7) | C15—C14—H14 | 119.9 |
S2—Sn—C31 | 87.83 (7) | C14—C15—C16 | 119.5 (3) |
C11—Sn—C21 | 104.11 (10) | C14—C15—H15 | 120.2 |
C11—Sn—C31 | 105.78 (10) | C16—C15—H15 | 120.2 |
C21—Sn—C31 | 115.55 (10) | C15—C16—C11 | 121.8 (3) |
C1—S1—Sn | 96.57 (10) | C15—C16—H16 | 119.1 |
C1—S2—Sn | 79.96 (10) | C11—C16—H16 | 119.1 |
C4—O1—C5 | 111.3 (3) | C22—C21—C26 | 118.1 (3) |
C1—N1—C2 | 121.6 (3) | C22—C21—Sn | 118.9 (2) |
C1—N1—C3 | 121.8 (3) | C26—C21—Sn | 122.9 (2) |
C2—N1—C3 | 116.4 (2) | C21—C22—C23 | 121.1 (3) |
N1—C1—S2 | 122.9 (2) | C21—C22—H22 | 119.4 |
N1—C1—S1 | 118.3 (2) | C23—C22—H22 | 119.4 |
S2—C1—S1 | 118.72 (16) | C24—C23—C22 | 119.7 (3) |
N1—C2—H2A | 109.5 | C24—C23—H23 | 120.2 |
N1—C2—H2B | 109.5 | C22—C23—H23 | 120.2 |
H2A—C2—H2B | 109.5 | C23—C24—C25 | 120.3 (3) |
N1—C2—H2C | 109.5 | C23—C24—H24 | 119.9 |
H2A—C2—H2C | 109.5 | C25—C24—H24 | 119.9 |
H2B—C2—H2C | 109.5 | C24—C25—C26 | 120.1 (3) |
N1—C3—C4 | 113.6 (3) | C24—C25—H25 | 120.0 |
N1—C3—H3A | 108.8 | C26—C25—H25 | 120.0 |
C4—C3—H3A | 108.8 | C25—C26—C21 | 120.7 (3) |
N1—C3—H3B | 108.8 | C25—C26—H26 | 119.6 |
C4—C3—H3B | 108.8 | C21—C26—H26 | 119.6 |
H3A—C3—H3B | 107.7 | C36—C31—C32 | 119.1 (2) |
O1—C4—C3 | 108.7 (3) | C36—C31—Sn | 117.20 (19) |
O1—C4—H4A | 109.9 | C32—C31—Sn | 123.70 (19) |
C3—C4—H4A | 109.9 | C33—C32—C31 | 119.9 (3) |
O1—C4—H4B | 109.9 | C33—C32—H32 | 120.0 |
C3—C4—H4B | 109.9 | C31—C32—H32 | 120.0 |
H4A—C4—H4B | 108.3 | C34—C33—C32 | 120.6 (3) |
O1—C5—H5A | 109.5 | C34—C33—H33 | 119.7 |
O1—C5—H5B | 109.5 | C32—C33—H33 | 119.7 |
H5A—C5—H5B | 109.5 | C35—C34—C33 | 120.0 (3) |
O1—C5—H5C | 109.5 | C35—C34—H34 | 120.0 |
H5A—C5—H5C | 109.5 | C33—C34—H34 | 120.0 |
H5B—C5—H5C | 109.5 | C34—C35—C36 | 119.7 (3) |
C12—C11—C16 | 117.5 (3) | C34—C35—H35 | 120.1 |
C12—C11—Sn | 123.0 (2) | C36—C35—H35 | 120.1 |
C16—C11—Sn | 119.5 (2) | C31—C36—C35 | 120.6 (3) |
C11—C12—C13 | 121.2 (3) | C31—C36—H36 | 119.7 |
C11—C12—H12 | 119.4 | C35—C36—H36 | 119.7 |
C13—C12—H12 | 119.4 | | |
| | | |
C2—N1—C1—S2 | −5.0 (4) | C12—C11—C16—C15 | 1.9 (5) |
C3—N1—C1—S2 | 179.3 (2) | Sn—C11—C16—C15 | −178.3 (2) |
C2—N1—C1—S1 | 175.7 (2) | C26—C21—C22—C23 | 1.3 (4) |
C3—N1—C1—S1 | 0.0 (4) | Sn—C21—C22—C23 | 177.9 (2) |
Sn—S2—C1—N1 | 175.2 (2) | C21—C22—C23—C24 | −1.1 (5) |
Sn—S2—C1—S1 | −5.46 (14) | C22—C23—C24—C25 | 0.0 (5) |
Sn—S1—C1—N1 | −174.0 (2) | C23—C24—C25—C26 | 0.8 (5) |
Sn—S1—C1—S2 | 6.62 (16) | C24—C25—C26—C21 | −0.6 (5) |
C1—N1—C3—C4 | 95.1 (3) | C22—C21—C26—C25 | −0.4 (4) |
C2—N1—C3—C4 | −80.8 (3) | Sn—C21—C26—C25 | −176.9 (2) |
C5—O1—C4—C3 | 178.7 (3) | C36—C31—C32—C33 | −0.4 (5) |
N1—C3—C4—O1 | 81.8 (3) | Sn—C31—C32—C33 | −179.7 (3) |
C16—C11—C12—C13 | −1.7 (5) | C31—C32—C33—C34 | −0.4 (5) |
Sn—C11—C12—C13 | 178.5 (2) | C32—C33—C34—C35 | 0.6 (6) |
C11—C12—C13—C14 | 0.5 (5) | C33—C34—C35—C36 | 0.0 (5) |
C12—C13—C14—C15 | 0.6 (5) | C32—C31—C36—C35 | 0.9 (4) |
C13—C14—C15—C16 | −0.4 (5) | Sn—C31—C36—C35 | −179.7 (2) |
C14—C15—C16—C11 | −0.9 (5) | C34—C35—C36—C31 | −0.8 (4) |
Hydrogen-bond geometry (Å, º) topCg1 is the centroid of the C21–C26 ring. |
D—H···A | D—H | H···A | D···A | D—H···A |
C35—H35···Cg1i | 0.95 | 2.99 | 3.760 (3) | 139 |
Symmetry code: (i) −x+1, −y, −z+1. |
Summary of short interatomic contacts (Å) in (I) topContact | Distance | Symmetry operation |
(I) | | |
H4B···H8A | 2.00 | -x, - y, 1 - z |
H5A···H6B | 2.21 | 1/2 - x, 1/2 - y, 1 - z |
H8B···H10B | 2.37 | -x, 1 - y, 1 - z |
H10B···H10B | 2.37 | -x, 1 - y, 1 - z |
(II) | | |
H14···H33 | 2.37 | 1 + x, 1 + y, z |
H16···H33 | 2.25 | x, 1 + y, z |
H22···H34 | 2.33 | x, 1 + y, z |
C1···H3B | 2.86 | -x, - y, 2 - z |
C14···H4A | 2.85 | 1 - x, 1 - y, 2 - z |
Percentage contributions of interatomic contacts to the Hirshfeld surface for (I) and (II) topContact | % contribution in (I) | % contribution in (II) |
H···H | 77.9 | 58.9 |
S···H/H···S | 12.2 | 7.3 |
C···H/H···C | 1.6 | 29.1 |
O···H/H···O | 7.9 | 2.5 |
N···H/H···N | 0.4 | 0.7 |
C···S/S···C | 0.0 | 1.3 |
S···O/O···S | 0.0 | 0.1 |
Sn···H/N···Sn | 0.0 | 0.1 |
Acknowledgements
We gratefully acknowledge the School of Chemical Science and Food Technology, Universiti Kebangsaan Malaysia, for providing the essential laboratory facilities. We would also like to thank the technical support received from the laboratory assistants of Faculty Science and Technology, Universiti Kebangsaan Malaysia. Intensity data were collected in the University of Malaya's crystallographic laboratory.
Funding information
This work was supported by grant No. GGP-2017-081 awarded by Universiti Kebangsaan Malaysia.
References
Addison, A. W., Rao, T. N., Reedijk, J., van Rijn, J. & Verschoor, G. C. (1984). J. Chem. Soc. Dalton Trans. pp. 1349–1356. CSD CrossRef Web of Science Google Scholar
Agilent (2015). CrysAlis PRO. Agilent Technologies Inc., Santa Clara, CA, USA. Google Scholar
Brandenburg, K. (2006). DIAMOND. Crystal Impact GbR, Bonn, Germany. Google Scholar
Farrugia, L. J. (2012). J. Appl. Cryst. 45, 849–854. Web of Science CrossRef CAS IUCr Journals Google Scholar
Ferreira, I. P., de Lima, G. M., Paniago, E. B., Rocha, W. R., Takahashi, J. A., Pinheiro, C. B. & Ardisson, J. D. (2012). Eur. J. Med. Chem. 58, 493–503. Web of Science CSD CrossRef CAS PubMed Google Scholar
Heard, P. J. (2005). Prog. Inorg. Chem. 53, 1–69. Web of Science CrossRef CAS Google Scholar
Hogarth, G. (2005). Prog. Inorg. Chem. 53, 71–561. Web of Science CrossRef CAS Google Scholar
Hogarth, G., Rainford-Brent, E. C.-R. C. R. & Richards, I. (2009). Inorg. Chim. Acta, 362, 1361–1364. Web of Science CSD CrossRef CAS Google Scholar
Jotani, M. M., Poplaukhin, P., Arman, H. D. & Tiekink, E. R. T. (2017). Z. Kristallogr. 232, 287–298. CAS Google Scholar
Khan, H., Badshah, A., Said, M., Murtaza, G., Ahmad, J., Jean-Claude, B. J., Todorova, M. & Butler, I. S. (2013). Appl. Organomet. Chem. 27, 387–395. Web of Science CSD CrossRef CAS Google Scholar
Khan, H., Badshah, A., Said, M., Murtaza, G., Sirajuddin, M., Ahmad, J. & Butler, I. S. (2016). Inorg. Chim. Acta, 447, 176–182. Web of Science CSD CrossRef CAS Google Scholar
McKinnon, J. J., Jayatilaka, D. & Spackman, M. A. (2007). Chem. Commun. pp. 3814–3816. Web of Science CrossRef Google Scholar
Mohamad, R., Awang, N., Jotani, M. M. & Tiekink, E. R. T. (2016). Acta Cryst. E72, 1130–1137. Web of Science CSD CrossRef IUCr Journals Google Scholar
Mohamad, R., Awang, N. & Kamaludin, N. F. (2016). Res. J. Pharm. Biol. Chem. Sci. 7, 1920–1925. CAS Google Scholar
Mohamad, R., Awang, N., Kamaludin, N. F. & Abu Bakar, N. F. (2016). Res. J. Pharm. Biol. Chem. Sci. 7, 1269–1274. CAS Google Scholar
Mohamad, R., Awang, N., Kamaludin, N. F., Jotani, M. M. & Tiekink, E. R. T. (2016). Acta Cryst. E72, 1480–1487. Web of Science CSD CrossRef IUCr Journals Google Scholar
Mohamad, R., Awang, N., Kamaludin, N. F., Jotani, M. M. & Tiekink, E. R. T. (2017). Acta Cryst. E73, 260–265. Web of Science CSD CrossRef IUCr Journals Google Scholar
Muthalib, A. F. A., Baba, I., Khaledi, H., Ali, H. M. & Tiekink, E. R. T. (2014). Z. Kristallogr. 229, 39–46. Google Scholar
Sheldrick, G. M. (2008). Acta Cryst. A64, 112–122. Web of Science CrossRef CAS IUCr Journals Google Scholar
Sheldrick, G. M. (2015). Acta Cryst. C71, 3–8. Web of Science CrossRef IUCr Journals Google Scholar
Spek, A. L. (2009). Acta Cryst. D65, 148–155. Web of Science CrossRef CAS IUCr Journals Google Scholar
Tan, Y. S., Halim, S. N. A. & Tiekink, E. R. T. (2016). Z. Kristallogr. 231, 113–126. CAS Google Scholar
Tiekink, E. R. T. (2008). Appl. Organomet. Chem. 22, 533–550. Web of Science CrossRef CAS Google Scholar
Westrip, S. P. (2010). J. Appl. Cryst. 43, 920–925. Web of Science CrossRef CAS IUCr Journals Google Scholar
Zaldi, N. B., Hussen, R. S. D., Lee, S. M., Halcovitch, N. R., Jotani, M. M. & Tiekink, E. R. T. (2017). Acta Cryst. E73, 842–848. Web of Science CSD CrossRef IUCr Journals Google Scholar
This is an open-access article distributed under the terms of the Creative Commons Attribution (CC-BY) Licence, which permits unrestricted use, distribution, and reproduction in any medium, provided the original authors and source are cited.
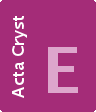 | CRYSTALLOGRAPHIC COMMUNICATIONS |
ISSN: 2056-9890
Open

access