1. Chemical context
A vast array of different dithiocarbamate anions, −S2CNRR′, has been prepared, which stems simply from the ability to alter the substituents in the starting amines used to prepare them. A key interest in dithiocarbamate compounds of both transition metals and main-group elements relates to their biological activity (Hogarth, 2012
). Of particular relevance to the present study is the anti-microbial potential exhibited by organotin dithiocarbamates (Tiekink, 2008
). In an on-going study of biological potential, organotin(IV) species have been complexed with two non-symmetric dithiocarbamate ligands, namely, with R = Me and R′ = n-Hex and CH2CH2Ph. Previously, similar species, i.e. R = benzyl and R′ = CH2CH2Ph (Mohamad, Awang, Kamaludin & Abu Bakar, 2016
; Segovia et al., 2002
) and R = Me and R′ = n-Bu (Segovia et al. 2002
) have been tested for their toxicity using a bioassay based on the inhibition of the growth of Escherichia coli with the latter compound being most toxic according to the EC50 value measured in vitro (Segovia et al., 2002
). These results gave rise to the suggestion that increasing the length of the alkyl chain leads to enhanced solubility/activity of the compound (Segovia et al., 2002
). Complementing these biological investigations (Mohamad, Awang, Kamaludin & Abu Bakar, 2016
; Mohamad, Awang & Kamaludin, 2016
) are structural studies of organotin dithiocarbamates (Mohamad, Awang, Jotani & Tiekink, 2016
; Mohamad, Awang, Kamaludin, Jotani et al., 2016
; Mohamad et al., 2017
, 2018
) and in continuation of the latter, herein, the crystal and molecular structures of (C6H5)3Sn[S2CN(Me)Hex] (I)
and (C6H5)3Sn[S2CN(CH3)CH2CH2Ph] (II)
are reported along with a Hirshfeld surface analysis to provide more details on the molecular packing.
2. Structural commentary
The tin atom in (I)
, Fig. 1
a, is coordinated by three ipso-carbon atoms along with a dithiocarbamate ligand. As seen from Table 1
, the dithiocarbamate ligand forms quite disparate Sn—S1, S2 bond lengths, with Δ(Sn—S) = (Sn—Slong – Sn—Sshort) being 0.64 Å. This asymmetry is confirmed in the differences in the C—S bond lengths with the C1—S1 bond associated with the short Sn—S1 contact, at 1.761 (4) Å, being significantly longer than the C1—S2 bond, i.e. 1.688 (4) Å, involving the weakly bound S2 atom. If the S2 atom is ignored, the coordination geometry about the tin atom is distorted C3S tetrahedral with the range of angles being 90.00 (11)°, for S1—Sn—C31, to 121.53 (10)°, for S1—Sn—C11. The wide angle clearly reflects the influence of the close approach of the S2 atom, Fig. 1
a and Table 1
. If the S2 atom is considered a significant bonding interaction, the resultant C3S2 donor set is almost perfectly intermediate between ideal square-pyramidal (SP) and trigonal–bipyramidal (TP). This is quantified in the value of τ = 0.52, which compares with the ideal values for SP and TP geometries of τ = 0.0 and 1.0, respectively (Addison et al., 1984
). In the latter description, the range of angles is wide with the S1—Sn—S2 chelate angle being acute [63.26 (3)°] and with the widest angle [152.54 (11)°] being for S2—Sn—C31. The n-hexyl chain is linear up to the terminal methyl group. Thus, the N1—C3—C4—C5, C3—C4—C5—C6 and C4—C5—C6—C7 torsion angles of 175.9 (4), 178.5 (4) and 178.9 (5)°, respectively, indicate + anti-periplanar descriptors but, that of C5—C6—C7—C8, i.e. −66.4 (8)°, indicative of a − syn-clinal disposition.
Parameter | (I) | (II) | Sn—S1 | 2.4672 (11) | 2.4636 (9) | Sn⋯S2 | 3.1113 (11) | 3.1066 (10) | C1—S1 | 1.761 (4) | 1.761 (4) | C1—S2 | 1.688 (4) | 1.678 (4) | C1—N1 | 1.330 (5) | 1.342 (5) | S1—Sn⋯S2 | 63.26 (3) | 63.42 (3) | S1—Sn⋯C11 | 121.53 (10) | 111.30 (9) | S1—Sn⋯C31 | 90.00 (11) | 92.68 (9) | C11—Sn—C21 | 114.88 (15) | 119.27 (13) | S2—Sn⋯C31 | 152.54 (11) | 155.43 (9) | | |
| Figure 1 The molecular structures of (a) (I) and (b) (II) , showing the atom-labelling schemes and displacement ellipsoids at the 50% probability level. |
The molecular structure of (II)
, Fig. 1
b, resembles closely that described for (I)
. Indeed, a comparison of the key bond lengths included in Table 1
show there are no chemically significant differences between the common parts of the molecules. In terms of bond angles, for a tetrahedral description, the range of angles in (II)
is smaller, by 2°, than in (I)
, again, not chemically significant. If the five-coordinate C3S2 description pertains, the value of τ = 0.60 indicates a distortion towards TP. The phenylethyl chain is kinked as seen in the N1—C3—C4—C5 and C3—C4—C5—C6 torsion angles of −175.8 (3) and 91.9 (5)°, respectively.
3. Supramolecular features
Tables 2
and 3
list the geometric parameters characterizing the intermolecular interactions operating in the crystals of (I)
and (II)
, respectively. The molecular packing of (I)
features centrosymmetric dimeric aggregates sustained by four phenyl-C—H⋯π(phenyl) interactions whereby all of the participating groups are derived from Sn-bound phenyl rings, Fig. 2
a. Such cooperative C—H⋯π(phenyl) embraces have been described for many phenyl-rich systems and in instances where six phenyl rings of two residues associate by edge-to-face interactions, i.e. a six-fold embrace, the energies of stabilization can resemble or even exceed that provided by strong conventional hydrogen bonding (Dance & Scudder, 2009
). The supramolecular dimers stack parallel to the b axis with no directional interactions between successive aggregates. Globally, columns pack into layers in the ab plane. The layers inter-digitate along the c axis, again without specific interactions between proximate residues, Fig. 2
b.
D—H⋯A | D—H | H⋯A | D⋯A | D—H⋯A | C32—H32⋯Cg1i | 0.95 | 2.88 | 3.630 (4) | 137 | C26—H26⋯Cg2i | 0.95 | 2.99 | 3.641 (5) | 127 | Symmetry code: (i) -x+1, -y+1, -z. | |
D—H⋯A | D—H | H⋯A | D⋯A | D—H⋯A | C2—H2B⋯Cg1i | 0.98 | 2.99 | 3.779 (5) | 138 | C14—H14⋯Cg2ii | 0.95 | 2.95 | 3.754 (5) | 143 | Symmetry codes: (i) -x+2, -y, -z+2; (ii) x, y-1, z. | |
| Figure 2 Molecular packing in the crystal of (I) : (a) supramolecular dimer sustained by a four-fold embrace of phenyl-C—H⋯π(phenyl) interactions shown as purple dashed lines (for clarity, the phenyl rings are shown as small spheres, the interacting phenyl rings are highlighted in purple and only the N-bound carbon atoms of the dithiocarbamate substituents are shown) and (b) a view of the unit-cell contents shown in projection down the b axis. |
The molecular packing of (II)
again features C—H⋯π interactions, as for (I)
, but with both methyl-H and Sn-bound-H hydrogen atoms as donors; the Sn-phenyl rings function as acceptors. As illustrated in Fig. 3
a, the C—H⋯π interactions sustain a supramolecular chain aligned along the b axis. The chains pack into the three-dimensional architecture without directional interactions between then, Fig. 3
b. As may be seen from Fig. 3
b, centrosymmetrically related Ph3Sn residues approach each other so as to form phenyl-embrace interactions as found in the molecular packing of (I)
, but none of the putative contacts are within the standard distance criteria assumed in PLATON (Spek, 2009
).
| Figure 3 Molecular packing in the crystal of (II) : (a) supramolecular chain sustained by C—H⋯π(phenyl) interactions shown as purple dashed lines and (b) a view of the unit-cell contents in projection down the b axis. One chain is highlighted in space-filling mode. |
4. Hirshfeld surface analysis
The Hirshfeld surface calculations for the triphenyltin dithiocarbamate derivatives (I)
and (II)
were performed in accord with recent work on related organotin dithiocarbamates (Mohamad et al., 2017
). Despite the similarity in composition, the structures of (I)
and (II)
exhibit different intermolecular environments because of the presence of different substituents in the respective dithiocarbamate ligands, i.e. n-hexyl in the former and phenylethyl in the latter. These differences are readily discerned from the differently shaped Hirshfeld surfaces mapped over dnorm for (I)
, Fig. 4
, and (II)
, Fig. 5
, which reflect the influence of short interatomic H⋯H and C⋯H/H⋯C contacts, Table 4
, and comparatively weak C—H⋯π interactions, Tables 2
and 3
.
Contact | Distance | Symmetry operation | (I) | | | H2C⋯H7B | 1.98 | x, − 1 + y, z | H2C⋯C7 | 2.67 | x, − 1 + y, z | H23⋯C32 | 2.57 | −x, − 1 + y, − 1 + z | H26⋯C33 | 2.70 | 1 − x, − 1 − y, − z | | | | (II) | | | H2A⋯H7 | 2.26 | 2 − x, + y, + z | H9⋯H23 | 2.29 | 1 + x, y, z | H2A⋯C7 | 2.68 | 2 − x, + y, + z | H2A⋯C8 | 2.74 | 2 − x, + y, + z | H2B⋯C11 | 2.70 | 2 − x, − y, 2 − z | H2B⋯C16 | 2.77 | 2 − x, − y, 2 − z | H22⋯C35 | 2.69 | 1 − x, − y, 2 − z | | |
| Figure 4 Views of Hirshfeld surface for (I) mapped over dnorm in the range −0.133 to +1.538 au. |
| Figure 5 Views of Hirshfeld surface for (II) mapped over dnorm in the range −0.075 to +1.363 au. |
The faint-red spots near the phenyl-C33 and H26 atoms in Fig. 4
a reflect the presence of a weak C—H⋯π interaction, as summarized in Table 4
. In both images of Fig. 4
, the bright-red spots appearing near Sn-bound phenyl atoms C32 and H23, methyl-H2C and n-hexyl atoms C7 and H7B are indicative of the short interatomic H⋯H and C⋯H/H⋯C contacts involving these atoms, as listed in Table 4
. The presence of similar intermolecular interactions in the crystal of (II)
cf. (I)
, but involving different atoms, is also characterized by bright and faint-red spots on the Hirshfeld surfaces mapped over dnorm in Fig. 5
. Thus, the C—H⋯π interaction is seen from the presence of bright-red spots near methyl-H2B and phenyl-C11 together with the pair of faint-red spots near the methyl-H2B and phenyl-C16 atoms in Fig. 5
a. The influence of other short interatomic C⋯H/H⋯C contacts summarized in Table 4
are viewed as diminutive and faint-red spots near the respective atoms in Fig. 5
a,b. The involvement of different atoms in the intermolecular interactions in the crystals of (I)
and (II)
is also confirmed from the views of their Hirshfeld surfaces mapped over electrostatic potential, Fig. 6
, through the appearance of blue and red regions corresponding to positive and negative electrostatic potentials around them. The different molecular environments about respective reference molecules are highlighted in Fig. 7
.
| Figure 6 Views of Hirshfeld surface mapped over the electrostatic potential (the red and blue regions represent negative and positive electrostatic potentials, respectively) for a molecule of: (a) (I) in the range ±0.041 au and (b) (II) in the range −0.033 to +0.049 au. |
| Figure 7 The immediate environment around reference molecules within dnorm-mapped Hirshfeld surfaces for (a) (I) and (b) (II) , highlighting short interatomic H⋯H and C⋯H/H⋯C contacts by yellow and blue dashed lines, respectively |
The distinct distribution of points in the overall two-dimensional fingerprint plots for (I)
and (II)
, Fig. 8
a, also highlight the different molecular environments for the two molecules. The significant contributions from H⋯H, C⋯H/H⋯C and S⋯H/H⋯S contacts to the Hirshfeld surfaces of both (I)
and (II)
are evident from Table 5
. The short interatomic H⋯H contact between the methyl-H2C and n-hexyl-H7B atoms in (I)
is viewed as a pair of closely spaced overlapping peaks with their tips at de + di ∼2.0 Å in the delineated plot (McKinnon et al., 2007
) Fig. 8
b. A pair of well separated short peaks at de + di ∼2.2 Å observed in the corresponding fingerprint plot for (II)
are due to the involvement of methyl-H2A and phenyl-H7, H9 and H23 atoms in comparatively weaker short interatomic H⋯H contacts, Table 4
. The pair of very thin and long forceps-like tips at de + di ∼2.6 Å in the fingerprint plot delineated into C⋯H/H⋯C contacts for (I)
, Fig. 8
c, is the result of a short interatomic contact between phenyl-C32 and -H23 atoms while the points corresponding to other short interatomic contacts are merged within the plot. The presence of a pair of twin forceps-like tips at de + di ∼ 2.7 Å in the C⋯H/H⋯C delineated plot for (II)
, Fig. 8
c, also indicates the involvement of methyl-H2A and -H2B, and phenyl-C7, -C8, -C11, -C16 and -C35 atoms in short interatomic contacts, Table 4
. Further, it is clear from the fingerprint plots delineated into S⋯H/H⋯S contacts, Fig. 8
d, that the pair of spikes at de + di ∼ 3.0 Å for (I)
show van der Waals contacts whereas the pair of peaks at de + di > 3.1 Å for (II)
show contacts farther than van der Waals separation. The other interatomic contacts summarized in Table 5
make a negligible contribution to their Hirshfeld surfaces.
Contact | Percentage contribution | | (I) | (II) | H⋯H | 66.1 | 57.8 | C⋯H/H⋯C | 25.6 | 33.7 | S⋯H/H⋯S | 7.6 | 7.6 | N⋯H/H⋯N | 0.4 | 0.6 | C⋯N/N⋯C | 0.2 | 0.0 | S⋯N/N⋯S | 0.1 | 0.0 | C⋯S/S⋯C | 0.0 | 0.3 | | |
| Figure 8 A comparison of the (a) full two-dimensional fingerprint plots for (I) and (II) , and the plots delineated into (b) H⋯H, (c) C⋯H/H⋯C and (d) S⋯H/H⋯S contacts. |
5. Database survey
The dithiocarbamate ligands reported in the present study are quite rare, despite the rather large number of crystal structures of dithiocarbamate ligands available in the crystallographic literature (Groom et al., 2016
). Thus, the N-hexyl-N-methyldithiocarbamate ligand reported in (I)
, i.e. dtcI, has been reported in the crystal structures of Ph2Sn(dtcI)2 (Ramasamy et al., 2013
), In(dtcI)3 (Park et al., 2003
), and in Bi(dtcI)3 and its 1:1 1,10-phenanthroline adduct (Monteiro et al., 2001
). The uniform motivation for these studies was for their evaluation as useful precursors for the deposition of heavy element sulfide nanomaterials. In terms of the molecular structures, no special features in the mode of coordination are noted in the tin (Tiekink, 2008
), indium (Heard, 2005
) and bismuth (Lai & Tiekink, 2007
) compounds. The N-methyl-N-phenylethyldithiocarbamate ligand, i.e. dtcII, has been reported only in its binary mercury(II) compound, i.e. Hg(dtcII)3 (Green et al., 2004
), and again, its study was motivated by the desire to generate β–HgS thin films and its structure confirms to expectation (Jotani et al., 2016
).
Reflecting the interest in organotin dithiocarbamates, including their biological activity, there are over 50 structures of general formula Ph3Sn(S2CNRR') in the Cambridge Structural Database (Groom et al., 2016
). Of these, seven are binuclear and are better represented as Ph3SnS2CN–R–NCS2SnPh3. In all, there are 56 independent coordination geometries and all conform to the same structural motif as described above for (I)
and (II)
. The average Sn—Sshort bond length is 2.47 Å and the average Sn—Slong bond length is 3.04 Å. This gives rise to an average Δ(Sn—S) of 0.57 Å. These values indicate the structures of (I)
and (II)
are outliers in that the values of Sn—Slong are generally longer than usually observed. An analysis of the available crystallographic data showed the shortest Sn—S1 bond length occurred in the structure of Ph3Sn(S2CNEt2) [(III); Hook et al. 1994
] while the longest was found for one of the independent tin centres in binuclear Ph3Sn[S2CN(CH2CH2)2C(H)(CH2)3C(H)(CH2CH2)2NCS2]SnPh3 [(IV); Ali et al., 2014
], i.e. spanning the range 2.43 to 2.52 Å, Table 6
. The shortest and longest of the Sn⋯S2 separations were found in Ph3Sn[S2CN(CH2Ph)CH2CH2Ph] [(V); Mohamad, Awang, Kamaludin, Jotani et al., 2016
] and for one of the two independent molecules of Ph3Sn{S2CN[CH2(3-pyridyl)]2} [(VI); Gupta et al., 2015
], i.e. spanning the range 2.91 to 3.22 Å, Table 6
. The lack of systematic variations in these structural parameters is borne out by the disparity of the cited bonds with the second tin centre of non-symmetric (IV) and the second independent molecule of (VI). Thus, the range of Δ(Sn—S) for all structures was 0.40 to 0.74, with the correlation coefficient from the plot of Sn—Sshort versus Sn—Slong being 0.52. Such a lack of correlation has often been noted in the structural chemistry of organotin dithiocarbamates and has been ascribed to the dictates of the molecular packing (Buntine et al., 1998
; Tiekink et al., 1999
; Muthalib et al., 2014
).
Compound | R | R′ | Sn—Sshort | Sn—Slong | Δ(Sn—S) | Reference | (III) | Et | Et | 2.429 (3) | 3.096 (3) | 0.67 | Hook et al. (1994 ) | (IV)a | (CH2CH2)2C(H)CH2CH2 | (CH2CH2)2C(H)CH2 | 2.521 (3) | 2.919 (3) | 0.40 | Ali et al. (2014 ) | | | | 2.4735 (10) | 2.9468 (10) | 0.47 | | (V) | CH2Ph | CH2CH2Ph | 2.4885 (5) | 2.9120 (5) | 0.42 | Mohamad, Awang, Kamaludin, Jotani et al. (2016 ) | (VI)b | CH2(3-pyridyl) | CH2(3-pyridyl) | 2.5165 (19) | 3.2209 (19) | 0.71 | Gupta et al. (2015 ) | | | | 2.4685 (19) | 3.0397 (19) | 0.57 | | Notes: (a) non-symmetric binuclear molecule; (b) two independent molecules in the asymmetric unit. | |
6. Synthesis and crystallization
All chemicals and solvents were used as purchased. The melting points were determined using an automated melting-point apparatus (MPA 120 EZ-Melt). C, H, N and S analyses were performed on a Leco CHNS-932 Elemental Analyzer. The IR spectra were obtained on a Perkin Elmer Spectrum GX from 4000 to 400 cm−1. NMR spectra were recorded in CDCl3 at room temperature on a Bruker AVANCE 400 111 HD.
Synthesis of triphenyltin(IV) N-hexyl-N-methyldithiocarbamate (I)
: N-hexyl-N-methylamine (Aldrich; 1.52 ml, 10 mmol) dissolved in ethanol (30 ml) was stirred at 277 K before a cold ethanolic solution of carbon disulfide (0.6 ml, 10 mmol) was added dropwise. The resulting mixture was stirred for 2 h. Then, triphenyltin(IV) chloride (Merck; 3.85 g, 10 mmol) dissolved in ethanol (25 ml) was added dropwise into the solution and stirring was continued for 2 h. The precipitate formed was filtered, washed with cold ethanol and dried. Recrystallization was achieved by dissolving the compound in a chloroform and ethanol mixture (1:1 v/v). This solution was allowed to slowly evaporate at room temperature yielding colourless slabs of (I)
. Yield: 52%, m.p. 364.6–365.4 K. Elemental analysis: calculated (%): C 57.8, H 5.8, N 2.6, S 11.9. Found (%): C 56.5, H 6.2, N 2.5, S 11.7. IR (KBr cm−1): 1429 ν(C—N), 983 ν(C—S), 559 ν(Sn—C), 425 ν(Sn—S). 1H NMR (CDCl3): δ 7.41–7.77 (15H, C6H5); 3.38 (2H, N—CH2); 3.42 (3H, N—CH3); 2.21 (2H, N—CH2CH2); 1.75 (2H, N—(CH2)2CH2); 1.59 (2H, N—(CH2)3CH2); 1.34 (2H, N—(CH2)4CH2); 0.92 (3H, hexyl—CH3). 13C NMR (CDCl3): δ 196.04 (NCS2); 128.52–142.53 (C-aromatic); 58.97 (NCH2); 43.79 (NCH3); 31.46 (N—CH2CH2); 26.98 [N—(CH2)2CH2]; 26.39 [N—(CH2)3CH2]; 22.6 [N—CH2)4CH2]; 14.06 (hexyl—CH3). 119Sn NMR (CDCl3): −187.56.
Synthesis of triphenyltin(IV) N-methyl-N-phenylethyldithiocarbamate (II)
: compound (II)
was prepared in essentially the same manner as for (I)
but using N-methyl-N-phenylethylamine (Aldrich; 1.45 ml, 10 mmol) in place of N-hexyl-N-methylamine. Recrystallization was achieved by dissolving the compound in a chloroform/ethanol mixture (1:2 v/v). Yield: 67%, m.p. 387.5–388.3 K. Elemental analysis: calculated (%): C 60.0, H 4.9, N 2.5, S 11.4. Found (%): C 57.9, H 5.3, N 2.8, S 11.2. IR (KBr cm−1): 1452 ν(C—N), 977 ν(C—S), 502 ν(Sn—C), 488 ν(Sn—S). 1H NMR (CDCl3): δ 7.43–7.77 (15H, Sn—C6H5); 7.24–7.35 [5H, N(CH2)2C6H5]; 4.06 (2H, NCH2); 3.36 (3H, NCH3); 3.09 (2H, NCH2CH2). 13C NMR (CDCl3): δ 196.61 (NCS2); 126.8–142.3 (C-aromatic); 60.25 (NCH2); 44.59 (NCH2CH2); 33.12 (N—CH3). 119Sn NMR (CDCl3) = −183.84.
7. Refinement
Crystal data, data collection and structure refinement details are summarized in Table 7
. Carbon-bound H atoms were placed in calculated positions (C—H = 0.95–0.99 Å) and were included in the refinement in the riding-model approximation, with Uiso(H) set to 1.2–1.5Ueq(C). For (I)
, the maximum and minimum residual electron density peaks of 1.75 and 1.51 e Å−3, respectively, are located 0.95 and 0.86 Å from the Sn atom. For (II)
, the maximum and minimum residual electron density peaks of 1.47 and 1.58 e Å−3, respectively, are located 0.96 and 0.68 Å from the C11 and Sn atoms, respectively.
| (I) | (II) | Crystal data | Chemical formula | [Sn(C6H5)3(C8H16NS2)] | [Sn(C6H5)3(C10H12NS2)] | Mr | 540.38 | 560.37 | Crystal system, space group | Triclinic, P![[\overline{1}]](teximages/hb7745fi10.gif) | Monoclinic, P21/c | Temperature (K) | 173 | 173 | a, b, c (Å) | 9.8590 (6), 10.4256 (5), 14.3960 (8) | 14.3682 (4), 9.4758 (2), 19.2747 (6) | α, β, γ (°) | 110.557 (5), 94.057 (5), 110.730 (5) | 90, 106.450 (3), 90 | V (Å3) | 1263.24 (13) | 2516.84 (12) | Z | 2 | 4 | Radiation type | Cu Kα | Cu Kα | μ (mm−1) | 9.67 | 9.73 | Crystal size (mm) | 0.30 × 0.20 × 0.05 | 0.10 × 0.10 × 0.05 | | Data collection | Diffractometer | Agilent Technologies SuperNova Dual diffractometer with Atlas detector | Agilent Technologies SuperNova Dual diffractometer with Atlas detector | Absorption correction | Multi-scan (CrysAlis PRO; Agilent, 2015 ) | Multi-scan (CrysAlis PRO; Agilent, 2015 ) | Tmin, Tmax | 0.204, 1.000 | 0.206, 1.000 | No. of measured, independent and observed [I > 2σ(I)] reflections | 8833, 5033, 4580 | 9741, 5054, 4431 | Rint | 0.057 | 0.040 | (sin θ/λ)max (Å−1) | 0.628 | 0.628 | | Refinement | R[F2 > 2σ(F2)], wR(F2), S | 0.043, 0.121, 1.06 | 0.039, 0.106, 1.02 | No. of reflections | 5033 | 5054 | No. of parameters | 273 | 290 | H-atom treatment | H-atom parameters constrained | H-atom parameters constrained | Δρmax, Δρmin (e Å−3) | 1.75, −1.51 | 1.47, −1.58 | Computer programs: CrysAlis PRO (Agilent, 2015 ), SIR92 (Altomare et al., 1993 ), SHELXL2014 (Sheldrick, 2015 ), ORTEP-3 for Windows (Farrugia, 2012 ), DIAMOND (Brandenburg, 2006 ) and publCIF (Westrip, 2010 ). | |
Supporting information
For both structures, data collection: CrysAlis PRO (Agilent, 2015); cell refinement: CrysAlis PRO (Agilent, 2015); data reduction: CrysAlis PRO (Agilent, 2015); program(s) used to solve structure: SIR92 (Altomare et al., 1993); program(s) used to refine structure: SHELXL2014 (Sheldrick, 2015); molecular graphics: ORTEP-3 for Windows (Farrugia, 2012) and DIAMOND (Brandenburg, 2006); software used to prepare material for publication: publCIF (Westrip, 2010).
(
N-Hexyl-
N-methyldithiocarbamato-
κ2S,
S')triphenyltin(IV) (I)
top Crystal data top [Sn(C6H5)3(C8H16NS2)] | Z = 2 |
Mr = 540.38 | F(000) = 552 |
Triclinic, P1 | Dx = 1.421 Mg m−3 |
a = 9.8590 (6) Å | Cu Kα radiation, λ = 1.54184 Å |
b = 10.4256 (5) Å | Cell parameters from 4558 reflections |
c = 14.3960 (8) Å | θ = 4.7–75.4° |
α = 110.557 (5)° | µ = 9.67 mm−1 |
β = 94.057 (5)° | T = 173 K |
γ = 110.730 (5)° | Prism, colourless |
V = 1263.24 (13) Å3 | 0.30 × 0.20 × 0.05 mm |
Data collection top Agilent Technologies SuperNova Dual diffractometer with Atlas detector | 5033 independent reflections |
Radiation source: SuperNova (Cu) X-ray Source | 4580 reflections with I > 2σ(I) |
Mirror monochromator | Rint = 0.057 |
ω scan | θmax = 75.4°, θmin = 4.7° |
Absorption correction: multi-scan (CrysAlis PRO; Agilent, 2015) | h = −11→12 |
Tmin = 0.204, Tmax = 1.000 | k = −12→13 |
8833 measured reflections | l = −17→17 |
Refinement top Refinement on F2 | 0 restraints |
Least-squares matrix: full | Hydrogen site location: inferred from neighbouring sites |
R[F2 > 2σ(F2)] = 0.043 | H-atom parameters constrained |
wR(F2) = 0.121 | w = 1/[σ2(Fo2) + (0.0811P)2] where P = (Fo2 + 2Fc2)/3 |
S = 1.06 | (Δ/σ)max = 0.001 |
5033 reflections | Δρmax = 1.75 e Å−3 |
273 parameters | Δρmin = −1.50 e Å−3 |
Special details top Geometry. All esds (except the esd in the dihedral angle between two l.s. planes) are estimated using the full covariance matrix. The cell esds are taken into account individually in the estimation of esds in distances, angles and torsion angles; correlations between esds in cell parameters are only used when they are defined by crystal symmetry. An approximate (isotropic) treatment of cell esds is used for estimating esds involving l.s. planes. |
Refinement. The maximum and minimum residual electron density peaks of 1.75 and 1.50 eÅ-3, respectively, were located 0.95 Å and 0.86 Å from the Sn atom. |
Fractional atomic coordinates and isotropic or equivalent isotropic displacement parameters (Å2) top | x | y | z | Uiso*/Ueq | |
Sn | 0.42003 (3) | 0.66365 (2) | 0.17688 (2) | 0.02940 (11) | |
S1 | 0.28273 (12) | 0.69634 (11) | 0.31417 (8) | 0.0359 (2) | |
S2 | 0.46733 (12) | 0.99031 (11) | 0.31126 (8) | 0.0375 (2) | |
N1 | 0.2964 (4) | 0.9556 (4) | 0.4439 (3) | 0.0361 (7) | |
C1 | 0.3470 (4) | 0.8930 (4) | 0.3645 (3) | 0.0311 (8) | |
C2 | 0.1843 (6) | 0.8691 (6) | 0.4837 (4) | 0.0466 (11) | |
H2A | 0.0966 | 0.7982 | 0.4288 | 0.070* | |
H2B | 0.1561 | 0.9368 | 0.5374 | 0.070* | |
H2C | 0.2248 | 0.8137 | 0.5119 | 0.070* | |
C3 | 0.3467 (5) | 1.1182 (5) | 0.4945 (3) | 0.0413 (10) | |
H3A | 0.4459 | 1.1674 | 0.4825 | 0.050* | |
H3B | 0.3566 | 1.1477 | 0.5688 | 0.050* | |
C4 | 0.2394 (5) | 1.1719 (5) | 0.4560 (4) | 0.0410 (9) | |
H4A | 0.1386 | 1.1175 | 0.4634 | 0.049* | |
H4B | 0.2351 | 1.1492 | 0.3828 | 0.049* | |
C5 | 0.2864 (6) | 1.3392 (5) | 0.5142 (4) | 0.0506 (11) | |
H5A | 0.3883 | 1.3936 | 0.5083 | 0.061* | |
H5B | 0.2880 | 1.3617 | 0.5871 | 0.061* | |
C6 | 0.1802 (7) | 1.3931 (6) | 0.4730 (4) | 0.0537 (12) | |
H6A | 0.1801 | 1.3713 | 0.4003 | 0.064* | |
H6B | 0.0781 | 1.3362 | 0.4774 | 0.064* | |
C7 | 0.2206 (9) | 1.5598 (7) | 0.5301 (5) | 0.0694 (17) | |
H7A | 0.3247 | 1.6157 | 0.5288 | 0.083* | |
H7B | 0.1564 | 1.5891 | 0.4932 | 0.083* | |
C8 | 0.2058 (9) | 1.6078 (7) | 0.6395 (5) | 0.0719 (18) | |
H8A | 0.1058 | 1.5468 | 0.6426 | 0.108* | |
H8B | 0.2218 | 1.7137 | 0.6672 | 0.108* | |
H8C | 0.2800 | 1.5941 | 0.6795 | 0.108* | |
C11 | 0.6597 (4) | 0.7469 (4) | 0.2096 (3) | 0.0304 (7) | |
C12 | 0.7510 (5) | 0.8927 (5) | 0.2260 (3) | 0.0379 (9) | |
H12 | 0.7089 | 0.9628 | 0.2292 | 0.045* | |
C13 | 0.9038 (5) | 0.9364 (5) | 0.2376 (4) | 0.0447 (10) | |
H13 | 0.9657 | 1.0367 | 0.2495 | 0.054* | |
C14 | 0.9665 (5) | 0.8351 (6) | 0.2318 (4) | 0.0454 (10) | |
H14 | 1.0710 | 0.8654 | 0.2394 | 0.054* | |
C15 | 0.8759 (5) | 0.6892 (6) | 0.2148 (4) | 0.0427 (10) | |
H15 | 0.9188 | 0.6195 | 0.2113 | 0.051* | |
C16 | 0.7237 (5) | 0.6441 (5) | 0.2028 (3) | 0.0356 (8) | |
H16 | 0.6622 | 0.5433 | 0.1900 | 0.043* | |
C21 | 0.3260 (4) | 0.7039 (4) | 0.0583 (3) | 0.0286 (7) | |
C22 | 0.1745 (5) | 0.6661 (5) | 0.0340 (4) | 0.0404 (9) | |
H22 | 0.1116 | 0.6201 | 0.0704 | 0.048* | |
C23 | 0.1134 (6) | 0.6942 (6) | −0.0422 (4) | 0.0513 (12) | |
H23 | 0.0091 | 0.6666 | −0.0583 | 0.062* | |
C24 | 0.2042 (6) | 0.7626 (6) | −0.0950 (4) | 0.0486 (11) | |
H24 | 0.1623 | 0.7819 | −0.1474 | 0.058* | |
C25 | 0.3532 (6) | 0.8021 (6) | −0.0721 (4) | 0.0491 (11) | |
H25 | 0.4154 | 0.8502 | −0.1079 | 0.059* | |
C26 | 0.4154 (5) | 0.7723 (5) | 0.0041 (3) | 0.0396 (9) | |
H26 | 0.5196 | 0.7990 | 0.0190 | 0.047* | |
C31 | 0.3442 (4) | 0.4284 (4) | 0.1449 (3) | 0.0300 (7) | |
C32 | 0.2560 (5) | 0.3130 (5) | 0.0524 (3) | 0.0359 (8) | |
H32 | 0.2296 | 0.3366 | −0.0024 | 0.043* | |
C33 | 0.2063 (5) | 0.1638 (5) | 0.0394 (4) | 0.0468 (11) | |
H33 | 0.1433 | 0.0870 | −0.0233 | 0.056* | |
C34 | 0.2475 (6) | 0.1261 (5) | 0.1167 (4) | 0.0519 (12) | |
H34 | 0.2146 | 0.0240 | 0.1071 | 0.062* | |
C35 | 0.3367 (6) | 0.2384 (6) | 0.2077 (4) | 0.0503 (12) | |
H35 | 0.3673 | 0.2135 | 0.2607 | 0.060* | |
C36 | 0.3824 (5) | 0.3874 (5) | 0.2229 (4) | 0.0423 (10) | |
H36 | 0.4408 | 0.4634 | 0.2872 | 0.051* | |
Atomic displacement parameters (Å2) top | U11 | U22 | U33 | U12 | U13 | U23 |
Sn | 0.02606 (15) | 0.03203 (15) | 0.03534 (16) | 0.01310 (11) | 0.00868 (10) | 0.01771 (11) |
S1 | 0.0372 (5) | 0.0344 (4) | 0.0426 (5) | 0.0156 (4) | 0.0165 (4) | 0.0201 (4) |
S2 | 0.0369 (5) | 0.0362 (5) | 0.0465 (5) | 0.0170 (4) | 0.0175 (4) | 0.0208 (4) |
N1 | 0.0326 (18) | 0.0422 (18) | 0.0386 (17) | 0.0188 (15) | 0.0119 (14) | 0.0177 (15) |
C1 | 0.0272 (18) | 0.0334 (18) | 0.0350 (18) | 0.0149 (15) | 0.0058 (15) | 0.0139 (15) |
C2 | 0.056 (3) | 0.049 (2) | 0.049 (2) | 0.027 (2) | 0.030 (2) | 0.027 (2) |
C3 | 0.041 (2) | 0.042 (2) | 0.039 (2) | 0.0205 (19) | 0.0047 (18) | 0.0101 (18) |
C4 | 0.038 (2) | 0.040 (2) | 0.049 (2) | 0.0189 (18) | 0.0124 (19) | 0.0191 (19) |
C5 | 0.053 (3) | 0.040 (2) | 0.060 (3) | 0.020 (2) | 0.017 (2) | 0.019 (2) |
C6 | 0.070 (3) | 0.052 (3) | 0.053 (3) | 0.032 (3) | 0.022 (2) | 0.028 (2) |
C7 | 0.095 (5) | 0.055 (3) | 0.084 (4) | 0.041 (3) | 0.044 (4) | 0.042 (3) |
C8 | 0.109 (6) | 0.062 (3) | 0.066 (4) | 0.049 (4) | 0.028 (4) | 0.033 (3) |
C11 | 0.0265 (18) | 0.0358 (18) | 0.0315 (17) | 0.0131 (15) | 0.0086 (14) | 0.0155 (15) |
C12 | 0.033 (2) | 0.038 (2) | 0.044 (2) | 0.0159 (17) | 0.0089 (17) | 0.0158 (17) |
C13 | 0.031 (2) | 0.045 (2) | 0.052 (2) | 0.0098 (18) | 0.0079 (19) | 0.018 (2) |
C14 | 0.026 (2) | 0.056 (3) | 0.049 (2) | 0.0161 (19) | 0.0061 (18) | 0.017 (2) |
C15 | 0.036 (2) | 0.056 (3) | 0.044 (2) | 0.028 (2) | 0.0103 (18) | 0.020 (2) |
C16 | 0.036 (2) | 0.042 (2) | 0.0357 (19) | 0.0205 (17) | 0.0106 (16) | 0.0192 (17) |
C21 | 0.0264 (18) | 0.0300 (16) | 0.0332 (17) | 0.0134 (14) | 0.0054 (14) | 0.0154 (14) |
C22 | 0.0256 (19) | 0.052 (2) | 0.050 (2) | 0.0162 (17) | 0.0109 (17) | 0.026 (2) |
C23 | 0.034 (2) | 0.070 (3) | 0.056 (3) | 0.026 (2) | 0.004 (2) | 0.027 (2) |
C24 | 0.056 (3) | 0.058 (3) | 0.044 (2) | 0.033 (2) | 0.005 (2) | 0.024 (2) |
C25 | 0.058 (3) | 0.060 (3) | 0.052 (3) | 0.031 (2) | 0.023 (2) | 0.039 (2) |
C26 | 0.030 (2) | 0.052 (2) | 0.047 (2) | 0.0187 (18) | 0.0131 (17) | 0.027 (2) |
C31 | 0.0247 (17) | 0.0306 (17) | 0.0366 (18) | 0.0113 (14) | 0.0093 (15) | 0.0151 (15) |
C32 | 0.030 (2) | 0.041 (2) | 0.042 (2) | 0.0147 (17) | 0.0176 (16) | 0.0204 (17) |
C33 | 0.042 (2) | 0.035 (2) | 0.049 (2) | 0.0056 (18) | 0.018 (2) | 0.0105 (19) |
C34 | 0.056 (3) | 0.033 (2) | 0.073 (3) | 0.017 (2) | 0.033 (3) | 0.027 (2) |
C35 | 0.059 (3) | 0.052 (3) | 0.063 (3) | 0.030 (2) | 0.026 (2) | 0.039 (2) |
C36 | 0.049 (3) | 0.037 (2) | 0.048 (2) | 0.0188 (19) | 0.010 (2) | 0.0238 (18) |
Geometric parameters (Å, º) top Sn—C21 | 2.123 (4) | C11—C16 | 1.404 (6) |
Sn—C31 | 2.156 (4) | C12—C13 | 1.389 (6) |
Sn—C11 | 2.159 (4) | C12—H12 | 0.9500 |
Sn—S1 | 2.4672 (11) | C13—C14 | 1.383 (7) |
Sn—S2 | 3.1113 (11) | C13—H13 | 0.9500 |
S1—C1 | 1.761 (4) | C14—C15 | 1.384 (7) |
S2—C1 | 1.688 (4) | C14—H14 | 0.9500 |
N1—C1 | 1.330 (5) | C15—C16 | 1.382 (6) |
N1—C2 | 1.453 (6) | C15—H15 | 0.9500 |
N1—C3 | 1.462 (6) | C16—H16 | 0.9500 |
C2—H2A | 0.9800 | C21—C22 | 1.386 (5) |
C2—H2B | 0.9800 | C21—C26 | 1.392 (6) |
C2—H2C | 0.9800 | C22—C23 | 1.382 (7) |
C3—C4 | 1.514 (6) | C22—H22 | 0.9500 |
C3—H3A | 0.9900 | C23—C24 | 1.383 (8) |
C3—H3B | 0.9900 | C23—H23 | 0.9500 |
C4—C5 | 1.518 (6) | C24—C25 | 1.358 (7) |
C4—H4A | 0.9900 | C24—H24 | 0.9500 |
C4—H4B | 0.9900 | C25—C26 | 1.398 (6) |
C5—C6 | 1.526 (7) | C25—H25 | 0.9500 |
C5—H5A | 0.9900 | C26—H26 | 0.9500 |
C5—H5B | 0.9900 | C31—C32 | 1.396 (6) |
C6—C7 | 1.521 (8) | C31—C36 | 1.405 (6) |
C6—H6A | 0.9900 | C32—C33 | 1.392 (6) |
C6—H6B | 0.9900 | C32—H32 | 0.9500 |
C7—C8 | 1.510 (9) | C33—C34 | 1.385 (8) |
C7—H7A | 0.9900 | C33—H33 | 0.9500 |
C7—H7B | 0.9900 | C34—C35 | 1.375 (8) |
C8—H8A | 0.9800 | C34—H34 | 0.9500 |
C8—H8B | 0.9800 | C35—C36 | 1.385 (6) |
C8—H8C | 0.9800 | C35—H35 | 0.9500 |
C11—C12 | 1.388 (6) | C36—H36 | 0.9500 |
| | | |
C21—Sn—C31 | 112.73 (14) | C7—C8—H8C | 109.5 |
C21—Sn—C11 | 114.88 (15) | H8A—C8—H8C | 109.5 |
C31—Sn—C11 | 104.73 (15) | H8B—C8—H8C | 109.5 |
C21—Sn—S1 | 109.94 (11) | C12—C11—C16 | 119.1 (4) |
C31—Sn—S1 | 90.00 (11) | C12—C11—Sn | 122.6 (3) |
C11—Sn—S1 | 121.53 (10) | C16—C11—Sn | 117.9 (3) |
C21—Sn—S2 | 84.08 (10) | C11—C12—C13 | 120.2 (4) |
C31—Sn—S2 | 152.54 (11) | C11—C12—H12 | 119.9 |
C11—Sn—S2 | 85.86 (11) | C13—C12—H12 | 119.9 |
S1—Sn—S2 | 63.26 (3) | C14—C13—C12 | 120.5 (4) |
C1—S1—Sn | 98.62 (14) | C14—C13—H13 | 119.7 |
C1—S2—Sn | 78.80 (14) | C12—C13—H13 | 119.7 |
C1—N1—C2 | 123.0 (4) | C13—C14—C15 | 119.5 (4) |
C1—N1—C3 | 121.5 (4) | C13—C14—H14 | 120.2 |
C2—N1—C3 | 115.4 (4) | C15—C14—H14 | 120.2 |
N1—C1—S2 | 124.0 (3) | C16—C15—C14 | 120.5 (4) |
N1—C1—S1 | 116.7 (3) | C16—C15—H15 | 119.7 |
S2—C1—S1 | 119.3 (2) | C14—C15—H15 | 119.7 |
N1—C2—H2A | 109.5 | C15—C16—C11 | 120.1 (4) |
N1—C2—H2B | 109.5 | C15—C16—H16 | 119.9 |
H2A—C2—H2B | 109.5 | C11—C16—H16 | 119.9 |
N1—C2—H2C | 109.5 | C22—C21—C26 | 118.0 (4) |
H2A—C2—H2C | 109.5 | C22—C21—Sn | 121.1 (3) |
H2B—C2—H2C | 109.5 | C26—C21—Sn | 120.9 (3) |
N1—C3—C4 | 111.7 (4) | C23—C22—C21 | 121.2 (4) |
N1—C3—H3A | 109.3 | C23—C22—H22 | 119.4 |
C4—C3—H3A | 109.3 | C21—C22—H22 | 119.4 |
N1—C3—H3B | 109.3 | C24—C23—C22 | 119.9 (4) |
C4—C3—H3B | 109.3 | C24—C23—H23 | 120.1 |
H3A—C3—H3B | 107.9 | C22—C23—H23 | 120.1 |
C3—C4—C5 | 111.4 (4) | C25—C24—C23 | 120.1 (4) |
C3—C4—H4A | 109.3 | C25—C24—H24 | 119.9 |
C5—C4—H4A | 109.4 | C23—C24—H24 | 119.9 |
C3—C4—H4B | 109.3 | C24—C25—C26 | 120.2 (5) |
C5—C4—H4B | 109.4 | C24—C25—H25 | 119.9 |
H4A—C4—H4B | 108.0 | C26—C25—H25 | 119.9 |
C4—C5—C6 | 111.1 (4) | C21—C26—C25 | 120.5 (4) |
C4—C5—H5A | 109.4 | C21—C26—H26 | 119.7 |
C6—C5—H5A | 109.4 | C25—C26—H26 | 119.7 |
C4—C5—H5B | 109.4 | C32—C31—C36 | 117.4 (4) |
C6—C5—H5B | 109.4 | C32—C31—Sn | 124.4 (3) |
H5A—C5—H5B | 108.0 | C36—C31—Sn | 118.2 (3) |
C7—C6—C5 | 113.2 (5) | C33—C32—C31 | 120.8 (4) |
C7—C6—H6A | 108.9 | C33—C32—H32 | 119.6 |
C5—C6—H6A | 108.9 | C31—C32—H32 | 119.6 |
C7—C6—H6B | 108.9 | C34—C33—C32 | 120.8 (4) |
C5—C6—H6B | 108.9 | C34—C33—H33 | 119.6 |
H6A—C6—H6B | 107.8 | C32—C33—H33 | 119.6 |
C8—C7—C6 | 115.3 (5) | C35—C34—C33 | 119.1 (4) |
C8—C7—H7A | 108.4 | C35—C34—H34 | 120.5 |
C6—C7—H7A | 108.4 | C33—C34—H34 | 120.5 |
C8—C7—H7B | 108.4 | C34—C35—C36 | 120.7 (5) |
C6—C7—H7B | 108.4 | C34—C35—H35 | 119.7 |
H7A—C7—H7B | 107.5 | C36—C35—H35 | 119.7 |
C7—C8—H8A | 109.5 | C35—C36—C31 | 121.2 (4) |
C7—C8—H8B | 109.5 | C35—C36—H36 | 119.4 |
H8A—C8—H8B | 109.5 | C31—C36—H36 | 119.4 |
| | | |
C2—N1—C1—S2 | 175.2 (3) | C14—C15—C16—C11 | −1.1 (7) |
C3—N1—C1—S2 | −2.3 (6) | C12—C11—C16—C15 | 1.5 (6) |
C2—N1—C1—S1 | −5.0 (5) | Sn—C11—C16—C15 | 174.7 (3) |
C3—N1—C1—S1 | 177.6 (3) | C26—C21—C22—C23 | 0.5 (7) |
Sn—S2—C1—N1 | 179.5 (4) | Sn—C21—C22—C23 | 179.0 (4) |
Sn—S2—C1—S1 | −0.32 (19) | C21—C22—C23—C24 | −0.7 (8) |
Sn—S1—C1—N1 | −179.4 (3) | C22—C23—C24—C25 | 0.0 (8) |
Sn—S1—C1—S2 | 0.4 (2) | C23—C24—C25—C26 | 0.8 (8) |
C1—N1—C3—C4 | 96.6 (5) | C22—C21—C26—C25 | 0.2 (7) |
C2—N1—C3—C4 | −81.0 (5) | Sn—C21—C26—C25 | −178.3 (4) |
N1—C3—C4—C5 | 175.9 (4) | C24—C25—C26—C21 | −0.9 (8) |
C3—C4—C5—C6 | 178.5 (4) | C36—C31—C32—C33 | 1.2 (6) |
C4—C5—C6—C7 | 178.9 (5) | Sn—C31—C32—C33 | −177.2 (3) |
C5—C6—C7—C8 | −66.4 (8) | C31—C32—C33—C34 | −2.3 (7) |
C16—C11—C12—C13 | −1.3 (6) | C32—C33—C34—C35 | 1.0 (8) |
Sn—C11—C12—C13 | −174.2 (3) | C33—C34—C35—C36 | 1.3 (8) |
C11—C12—C13—C14 | 0.7 (7) | C34—C35—C36—C31 | −2.5 (8) |
C12—C13—C14—C15 | −0.3 (7) | C32—C31—C36—C35 | 1.2 (7) |
C13—C14—C15—C16 | 0.5 (7) | Sn—C31—C36—C35 | 179.6 (4) |
Hydrogen-bond geometry (Å, º) topCg1 and Cg2 are the centroids of the (C11–C16) and (C31–C36) rings, respectively. |
D—H···A | D—H | H···A | D···A | D—H···A |
C32—H32···Cg1i | 0.95 | 2.88 | 3.630 (4) | 137 |
C26—H26···Cg2i | 0.95 | 2.99 | 3.641 (5) | 127 |
Symmetry code: (i) −x+1, −y+1, −z. |
[
N-Methyl-
N-(2-phenylethyl)dithiocarbamato-
κ2S,
S']triphenyltin(IV) (II)
top Crystal data top [Sn(C6H5)3(C10H12NS2)] | F(000) = 1136 |
Mr = 560.37 | Dx = 1.479 Mg m−3 |
Monoclinic, P21/c | Cu Kα radiation, λ = 1.54184 Å |
a = 14.3682 (4) Å | Cell parameters from 4369 reflections |
b = 9.4758 (2) Å | θ = 4.8–75.1° |
c = 19.2747 (6) Å | µ = 9.73 mm−1 |
β = 106.450 (3)° | T = 173 K |
V = 2516.84 (12) Å3 | Prism, colourless |
Z = 4 | 0.10 × 0.10 × 0.05 mm |
Data collection top Agilent Technologies SuperNova Dual diffractometer with Atlas detector | 5054 independent reflections |
Radiation source: SuperNova (Cu) X-ray Source | 4431 reflections with I > 2σ(I) |
Mirror monochromator | Rint = 0.040 |
Detector resolution: 10.4041 pixels mm-1 | θmax = 75.6°, θmin = 4.8° |
ω scan | h = −17→13 |
Absorption correction: multi-scan (CrysAlis PRO; Agilent, 2015) | k = −11→7 |
Tmin = 0.206, Tmax = 1.000 | l = −22→24 |
9741 measured reflections | |
Refinement top Refinement on F2 | 0 restraints |
Least-squares matrix: full | Hydrogen site location: inferred from neighbouring sites |
R[F2 > 2σ(F2)] = 0.039 | H-atom parameters constrained |
wR(F2) = 0.106 | w = 1/[σ2(Fo2) + (0.0648P)2] where P = (Fo2 + 2Fc2)/3 |
S = 1.02 | (Δ/σ)max = 0.003 |
5054 reflections | Δρmax = 1.47 e Å−3 |
290 parameters | Δρmin = −1.58 e Å−3 |
Special details top Geometry. All esds (except the esd in the dihedral angle between two l.s. planes) are estimated using the full covariance matrix. The cell esds are taken into account individually in the estimation of esds in distances, angles and torsion angles; correlations between esds in cell parameters are only used when they are defined by crystal symmetry. An approximate (isotropic) treatment of cell esds is used for estimating esds involving l.s. planes. |
Refinement. The maximum and minimum residual electron density peaks of 1.47 and 1.58 eÅ-3, respectively, were located 0.96 Å and 0.68 Å from the C11 and Sn atoms, respectively. |
Fractional atomic coordinates and isotropic or equivalent isotropic displacement parameters (Å2) top | x | y | z | Uiso*/Ueq | |
Sn | 0.68172 (2) | 0.06519 (2) | 0.97084 (2) | 0.02404 (9) | |
S1 | 0.84111 (6) | 0.17866 (9) | 0.98902 (5) | 0.0307 (2) | |
S2 | 0.78587 (7) | 0.01259 (10) | 0.85224 (5) | 0.0350 (2) | |
N1 | 0.9625 (2) | 0.1189 (3) | 0.91290 (17) | 0.0320 (7) | |
C1 | 0.8706 (3) | 0.1012 (4) | 0.91495 (19) | 0.0279 (7) | |
C2 | 1.0356 (3) | 0.1925 (4) | 0.9697 (2) | 0.0369 (8) | |
H2A | 1.0283 | 0.2946 | 0.9619 | 0.055* | |
H2B | 1.1005 | 0.1636 | 0.9683 | 0.055* | |
H2C | 1.0270 | 0.1687 | 1.0170 | 0.055* | |
C3 | 0.9948 (3) | 0.0678 (4) | 0.8520 (2) | 0.0344 (9) | |
H3A | 0.9483 | −0.0036 | 0.8248 | 0.041* | |
H3B | 1.0589 | 0.0216 | 0.8705 | 0.041* | |
C4 | 1.0026 (3) | 0.1877 (4) | 0.8013 (2) | 0.0427 (10) | |
H4A | 0.9398 | 0.2383 | 0.7856 | 0.051* | |
H4B | 1.0527 | 0.2555 | 0.8275 | 0.051* | |
C5 | 1.0287 (3) | 0.1338 (4) | 0.7357 (2) | 0.0353 (8) | |
C6 | 0.9574 (3) | 0.0996 (4) | 0.6730 (2) | 0.0386 (9) | |
H6 | 0.8911 | 0.1128 | 0.6709 | 0.046* | |
C7 | 0.9812 (3) | 0.0467 (4) | 0.6137 (2) | 0.0400 (9) | |
H7 | 0.9311 | 0.0239 | 0.5712 | 0.048* | |
C8 | 1.0764 (3) | 0.0264 (5) | 0.6150 (2) | 0.0401 (9) | |
H8 | 1.0924 | −0.0106 | 0.5740 | 0.048* | |
C9 | 1.1483 (3) | 0.0605 (6) | 0.6769 (3) | 0.0513 (13) | |
H9 | 1.2144 | 0.0472 | 0.6784 | 0.062* | |
C10 | 1.1254 (3) | 0.1139 (6) | 0.7368 (2) | 0.0469 (11) | |
H10 | 1.1759 | 0.1372 | 0.7790 | 0.056* | |
C11 | 0.6968 (2) | −0.1552 (3) | 0.9966 (2) | 0.0245 (7) | |
C12 | 0.6890 (3) | −0.1965 (4) | 1.0642 (2) | 0.0311 (8) | |
H12 | 0.6760 | −0.1278 | 1.0961 | 0.037* | |
C13 | 0.7002 (3) | −0.3379 (4) | 1.0855 (2) | 0.0392 (9) | |
H13 | 0.6963 | −0.3644 | 1.1321 | 0.047* | |
C14 | 0.7168 (3) | −0.4394 (4) | 1.0389 (3) | 0.0377 (9) | |
H14 | 0.7242 | −0.5356 | 1.0533 | 0.045* | |
C15 | 0.7227 (3) | −0.4001 (4) | 0.9714 (2) | 0.0344 (8) | |
H15 | 0.7331 | −0.4699 | 0.9390 | 0.041* | |
C16 | 0.7137 (3) | −0.2591 (4) | 0.9504 (2) | 0.0308 (8) | |
H16 | 0.7190 | −0.2334 | 0.9040 | 0.037* | |
C21 | 0.5674 (2) | 0.1332 (3) | 0.88077 (18) | 0.0238 (7) | |
C22 | 0.5054 (3) | 0.0368 (4) | 0.8369 (2) | 0.0351 (8) | |
H22 | 0.5164 | −0.0613 | 0.8457 | 0.042* | |
C23 | 0.4274 (3) | 0.0811 (5) | 0.7804 (2) | 0.0424 (10) | |
H23 | 0.3857 | 0.0136 | 0.7507 | 0.051* | |
C24 | 0.4107 (3) | 0.2222 (5) | 0.7676 (2) | 0.0414 (10) | |
H24 | 0.3568 | 0.2528 | 0.7294 | 0.050* | |
C25 | 0.4718 (4) | 0.3196 (4) | 0.8099 (2) | 0.0449 (10) | |
H25 | 0.4606 | 0.4174 | 0.8003 | 0.054* | |
C26 | 0.5496 (3) | 0.2767 (4) | 0.8665 (2) | 0.0366 (9) | |
H26 | 0.5912 | 0.3450 | 0.8957 | 0.044* | |
C31 | 0.6570 (2) | 0.1695 (4) | 1.06344 (19) | 0.0262 (7) | |
C32 | 0.7271 (3) | 0.1666 (4) | 1.1310 (2) | 0.0311 (8) | |
H32 | 0.7863 | 0.1174 | 1.1362 | 0.037* | |
C33 | 0.7110 (3) | 0.2349 (4) | 1.1904 (2) | 0.0331 (8) | |
H33 | 0.7588 | 0.2309 | 1.2360 | 0.040* | |
C34 | 0.6260 (3) | 0.3084 (4) | 1.1836 (2) | 0.0328 (8) | |
H34 | 0.6152 | 0.3545 | 1.2244 | 0.039* | |
C35 | 0.5566 (3) | 0.3150 (4) | 1.1174 (2) | 0.0348 (8) | |
H35 | 0.4986 | 0.3670 | 1.1125 | 0.042* | |
C36 | 0.5718 (3) | 0.2455 (4) | 1.0579 (2) | 0.0284 (7) | |
H36 | 0.5232 | 0.2497 | 1.0127 | 0.034* | |
Atomic displacement parameters (Å2) top | U11 | U22 | U33 | U12 | U13 | U23 |
Sn | 0.02450 (14) | 0.02123 (14) | 0.02577 (14) | 0.00113 (8) | 0.00611 (10) | −0.00044 (8) |
S1 | 0.0308 (4) | 0.0320 (4) | 0.0324 (4) | −0.0046 (3) | 0.0139 (4) | −0.0054 (3) |
S2 | 0.0352 (5) | 0.0397 (5) | 0.0307 (5) | −0.0032 (4) | 0.0104 (4) | −0.0035 (4) |
N1 | 0.0303 (16) | 0.0367 (17) | 0.0309 (16) | 0.0030 (13) | 0.0117 (14) | 0.0010 (13) |
C1 | 0.0307 (19) | 0.0294 (17) | 0.0279 (17) | 0.0023 (15) | 0.0152 (15) | 0.0038 (14) |
C2 | 0.0300 (19) | 0.044 (2) | 0.036 (2) | 0.0008 (17) | 0.0076 (17) | 0.0023 (17) |
C3 | 0.031 (2) | 0.037 (2) | 0.042 (2) | 0.0049 (15) | 0.0213 (18) | 0.0011 (16) |
C4 | 0.056 (3) | 0.038 (2) | 0.039 (2) | 0.0026 (19) | 0.022 (2) | 0.0010 (17) |
C5 | 0.043 (2) | 0.0305 (19) | 0.035 (2) | 0.0003 (16) | 0.0158 (18) | 0.0041 (15) |
C6 | 0.033 (2) | 0.039 (2) | 0.044 (2) | 0.0091 (17) | 0.0105 (19) | 0.0015 (18) |
C7 | 0.037 (2) | 0.041 (2) | 0.038 (2) | 0.0040 (17) | 0.0031 (19) | −0.0035 (17) |
C8 | 0.041 (2) | 0.049 (2) | 0.033 (2) | −0.0006 (19) | 0.0169 (18) | 0.0011 (18) |
C9 | 0.029 (2) | 0.086 (4) | 0.041 (3) | −0.006 (2) | 0.015 (2) | 0.001 (2) |
C10 | 0.036 (2) | 0.073 (3) | 0.031 (2) | −0.011 (2) | 0.0091 (18) | −0.003 (2) |
C11 | 0.0182 (15) | 0.0191 (15) | 0.0334 (18) | 0.0001 (12) | 0.0029 (14) | 0.0021 (13) |
C12 | 0.0356 (19) | 0.0228 (16) | 0.038 (2) | 0.0012 (14) | 0.0153 (17) | 0.0001 (14) |
C13 | 0.045 (2) | 0.034 (2) | 0.042 (2) | 0.0025 (18) | 0.0184 (19) | 0.0113 (17) |
C14 | 0.037 (2) | 0.0227 (18) | 0.057 (3) | 0.0018 (14) | 0.020 (2) | 0.0058 (16) |
C15 | 0.031 (2) | 0.0254 (17) | 0.048 (2) | −0.0052 (15) | 0.0145 (18) | −0.0101 (16) |
C16 | 0.0303 (18) | 0.0284 (17) | 0.0335 (19) | −0.0042 (15) | 0.0087 (16) | −0.0026 (15) |
C21 | 0.0246 (16) | 0.0227 (16) | 0.0241 (16) | 0.0022 (13) | 0.0067 (14) | 0.0012 (12) |
C22 | 0.040 (2) | 0.0238 (17) | 0.040 (2) | −0.0031 (16) | 0.0078 (18) | 0.0025 (15) |
C23 | 0.033 (2) | 0.051 (3) | 0.035 (2) | −0.0049 (18) | −0.0032 (18) | −0.0001 (18) |
C24 | 0.038 (2) | 0.055 (3) | 0.030 (2) | 0.0156 (19) | 0.0076 (18) | 0.0075 (18) |
C25 | 0.060 (3) | 0.030 (2) | 0.042 (2) | 0.0186 (19) | 0.010 (2) | 0.0098 (17) |
C26 | 0.048 (2) | 0.0220 (17) | 0.034 (2) | 0.0027 (16) | 0.0033 (18) | 0.0005 (14) |
C31 | 0.0257 (17) | 0.0235 (16) | 0.0295 (17) | −0.0039 (14) | 0.0080 (15) | −0.0034 (13) |
C32 | 0.0314 (18) | 0.0293 (18) | 0.0326 (19) | 0.0040 (15) | 0.0092 (16) | −0.0026 (15) |
C33 | 0.0330 (19) | 0.0358 (19) | 0.0277 (18) | −0.0020 (16) | 0.0043 (16) | −0.0078 (15) |
C34 | 0.037 (2) | 0.0248 (17) | 0.041 (2) | −0.0015 (15) | 0.0173 (17) | −0.0106 (15) |
C35 | 0.0273 (18) | 0.0295 (18) | 0.050 (2) | 0.0024 (15) | 0.0149 (17) | −0.0056 (16) |
C36 | 0.0216 (16) | 0.0292 (17) | 0.0318 (18) | −0.0008 (14) | 0.0032 (14) | −0.0025 (14) |
Geometric parameters (Å, º) top Sn—C21 | 2.125 (3) | C12—C13 | 1.397 (5) |
Sn—C11 | 2.143 (3) | C12—H12 | 0.9500 |
Sn—C31 | 2.156 (3) | C13—C14 | 1.382 (6) |
Sn—S1 | 2.4636 (9) | C13—H13 | 0.9500 |
Sn—S2 | 3.1066 (10) | C14—C15 | 1.379 (6) |
S1—C1 | 1.761 (4) | C14—H14 | 0.9500 |
S2—C1 | 1.678 (4) | C15—C16 | 1.392 (5) |
N1—C1 | 1.342 (5) | C15—H15 | 0.9500 |
N1—C2 | 1.462 (5) | C16—H16 | 0.9500 |
N1—C3 | 1.462 (5) | C21—C22 | 1.384 (5) |
C2—H2A | 0.9800 | C21—C26 | 1.396 (5) |
C2—H2B | 0.9800 | C22—C23 | 1.389 (6) |
C2—H2C | 0.9800 | C22—H22 | 0.9500 |
C3—C4 | 1.524 (5) | C23—C24 | 1.369 (6) |
C3—H3A | 0.9900 | C23—H23 | 0.9500 |
C3—H3B | 0.9900 | C24—C25 | 1.373 (6) |
C4—C5 | 1.505 (5) | C24—H24 | 0.9500 |
C4—H4A | 0.9900 | C25—C26 | 1.383 (6) |
C4—H4B | 0.9900 | C25—H25 | 0.9500 |
C5—C6 | 1.384 (6) | C26—H26 | 0.9500 |
C5—C10 | 1.396 (6) | C31—C32 | 1.403 (5) |
C6—C7 | 1.378 (6) | C31—C36 | 1.398 (5) |
C6—H6 | 0.9500 | C32—C33 | 1.391 (5) |
C7—C8 | 1.375 (6) | C32—H32 | 0.9500 |
C7—H7 | 0.9500 | C33—C34 | 1.379 (5) |
C8—C9 | 1.376 (7) | C33—H33 | 0.9500 |
C8—H8 | 0.9500 | C34—C35 | 1.380 (6) |
C9—C10 | 1.383 (6) | C34—H34 | 0.9500 |
C9—H9 | 0.9500 | C35—C36 | 1.392 (5) |
C10—H10 | 0.9500 | C35—H35 | 0.9500 |
C11—C12 | 1.397 (5) | C36—H36 | 0.9500 |
C11—C16 | 1.393 (5) | | |
| | | |
C21—Sn—C11 | 119.27 (13) | C12—C11—C16 | 118.2 (3) |
C21—Sn—C31 | 105.46 (13) | C12—C11—Sn | 117.4 (3) |
C11—Sn—C31 | 106.56 (13) | C16—C11—Sn | 124.4 (3) |
C21—Sn—S1 | 117.16 (9) | C11—C12—C13 | 120.7 (3) |
C11—Sn—S1 | 111.30 (9) | C11—C12—H12 | 119.7 |
C31—Sn—S1 | 92.68 (9) | C13—C12—H12 | 119.7 |
C21—Sn—S2 | 82.51 (9) | C14—C13—C12 | 120.2 (4) |
C11—Sn—S2 | 88.60 (10) | C14—C13—H13 | 119.9 |
C31—Sn—S2 | 155.43 (9) | C12—C13—H13 | 119.9 |
S1—Sn—S2 | 63.42 (3) | C15—C14—C13 | 119.6 (3) |
C1—S1—Sn | 97.50 (13) | C15—C14—H14 | 120.2 |
C1—S2—Sn | 78.01 (12) | C13—C14—H14 | 120.2 |
C1—N1—C2 | 122.8 (3) | C14—C15—C16 | 120.5 (4) |
C1—N1—C3 | 121.4 (3) | C14—C15—H15 | 119.7 |
C2—N1—C3 | 115.8 (3) | C16—C15—H15 | 119.7 |
N1—C1—S2 | 124.1 (3) | C15—C16—C11 | 120.7 (4) |
N1—C1—S1 | 115.9 (3) | C15—C16—H16 | 119.6 |
S2—C1—S1 | 120.0 (2) | C11—C16—H16 | 119.6 |
N1—C2—H2A | 109.5 | C22—C21—C26 | 118.2 (3) |
N1—C2—H2B | 109.5 | C22—C21—Sn | 120.9 (3) |
H2A—C2—H2B | 109.5 | C26—C21—Sn | 120.8 (3) |
N1—C2—H2C | 109.5 | C23—C22—C21 | 121.1 (4) |
H2A—C2—H2C | 109.5 | C23—C22—H22 | 119.4 |
H2B—C2—H2C | 109.5 | C21—C22—H22 | 119.4 |
N1—C3—C4 | 111.5 (3) | C24—C23—C22 | 119.9 (4) |
N1—C3—H3A | 109.3 | C24—C23—H23 | 120.1 |
C4—C3—H3A | 109.3 | C22—C23—H23 | 120.1 |
N1—C3—H3B | 109.3 | C23—C24—C25 | 120.0 (4) |
C4—C3—H3B | 109.3 | C23—C24—H24 | 120.0 |
H3A—C3—H3B | 108.0 | C25—C24—H24 | 120.0 |
C5—C4—C3 | 111.5 (3) | C24—C25—C26 | 120.6 (4) |
C5—C4—H4A | 109.3 | C24—C25—H25 | 119.7 |
C3—C4—H4A | 109.3 | C26—C25—H25 | 119.7 |
C5—C4—H4B | 109.3 | C25—C26—C21 | 120.3 (4) |
C3—C4—H4B | 109.3 | C25—C26—H26 | 119.9 |
H4A—C4—H4B | 108.0 | C21—C26—H26 | 119.9 |
C6—C5—C10 | 118.0 (4) | C32—C31—C36 | 117.6 (3) |
C6—C5—C4 | 120.8 (4) | C32—C31—Sn | 121.1 (2) |
C10—C5—C4 | 121.2 (4) | C36—C31—Sn | 121.3 (3) |
C7—C6—C5 | 120.9 (4) | C31—C32—C33 | 120.8 (3) |
C7—C6—H6 | 119.6 | C31—C32—H32 | 119.6 |
C5—C6—H6 | 119.6 | C33—C32—H32 | 119.6 |
C6—C7—C8 | 121.0 (4) | C34—C33—C32 | 120.3 (4) |
C6—C7—H7 | 119.5 | C34—C33—H33 | 119.8 |
C8—C7—H7 | 119.5 | C32—C33—H33 | 119.8 |
C7—C8—C9 | 118.8 (4) | C35—C34—C33 | 120.0 (3) |
C7—C8—H8 | 120.6 | C35—C34—H34 | 120.0 |
C9—C8—H8 | 120.6 | C33—C34—H34 | 120.0 |
C8—C9—C10 | 120.8 (4) | C34—C35—C36 | 120.0 (3) |
C8—C9—H9 | 119.6 | C34—C35—H35 | 120.0 |
C10—C9—H9 | 119.6 | C36—C35—H35 | 120.0 |
C9—C10—C5 | 120.5 (4) | C35—C36—C31 | 121.2 (3) |
C9—C10—H10 | 119.7 | C35—C36—H36 | 119.4 |
C5—C10—H10 | 119.7 | C31—C36—H36 | 119.4 |
| | | |
C2—N1—C1—S2 | −177.8 (3) | C11—C12—C13—C14 | −1.5 (6) |
C3—N1—C1—S2 | 3.7 (5) | C12—C13—C14—C15 | 0.2 (6) |
C2—N1—C1—S1 | 2.4 (5) | C13—C14—C15—C16 | 1.1 (6) |
C3—N1—C1—S1 | −176.0 (3) | C14—C15—C16—C11 | −1.1 (6) |
Sn—S2—C1—N1 | 171.3 (3) | C12—C11—C16—C15 | −0.2 (5) |
Sn—S2—C1—S1 | −9.00 (18) | Sn—C11—C16—C15 | 179.9 (3) |
Sn—S1—C1—N1 | −169.0 (3) | C26—C21—C22—C23 | −0.2 (6) |
Sn—S1—C1—S2 | 11.2 (2) | Sn—C21—C22—C23 | 176.5 (3) |
C1—N1—C3—C4 | 102.6 (4) | C21—C22—C23—C24 | −0.3 (7) |
C2—N1—C3—C4 | −76.0 (4) | C22—C23—C24—C25 | 0.9 (7) |
N1—C3—C4—C5 | −175.8 (3) | C23—C24—C25—C26 | −1.1 (7) |
C3—C4—C5—C6 | 91.9 (5) | C24—C25—C26—C21 | 0.5 (7) |
C3—C4—C5—C10 | −86.7 (5) | C22—C21—C26—C25 | 0.1 (6) |
C10—C5—C6—C7 | 0.4 (6) | Sn—C21—C26—C25 | −176.6 (3) |
C4—C5—C6—C7 | −178.2 (4) | C36—C31—C32—C33 | 1.2 (5) |
C5—C6—C7—C8 | 0.0 (7) | Sn—C31—C32—C33 | 179.6 (3) |
C6—C7—C8—C9 | −0.3 (7) | C31—C32—C33—C34 | −0.9 (6) |
C7—C8—C9—C10 | 0.2 (7) | C32—C33—C34—C35 | −0.3 (6) |
C8—C9—C10—C5 | 0.2 (8) | C33—C34—C35—C36 | 1.1 (6) |
C6—C5—C10—C9 | −0.5 (7) | C34—C35—C36—C31 | −0.7 (6) |
C4—C5—C10—C9 | 178.1 (4) | C32—C31—C36—C35 | −0.4 (5) |
C16—C11—C12—C13 | 1.5 (5) | Sn—C31—C36—C35 | −178.8 (3) |
Sn—C11—C12—C13 | −178.6 (3) | | |
Hydrogen-bond geometry (Å, º) topHydrogen-bond geometry (Å, °) for (I). Cg1 and Cg2 are the ring centroids of the (C11–C16) and (C31–C36) rings, respectively. |
D—H···A | D—H | H···A | D···A | D—H···A |
C2—H2B···Cg1i | 0.98 | 2.99 | 3.779 (5) | 138 |
C14—H14···Cg2ii | 0.95 | 2.95 | 3.754 (5) | 143 |
Symmetry codes: (i) −x+2, −y, −z+2; (ii) x, y−1, z. |
Selected interatomic parameters (Å, °) for (I) and (II) topParameter | (I) | (II) |
Sn—S1 | 2.4672 (11) | 2.4636 (9) |
Sn···S2 | 3.1113 (11) | 3.1066 (10) |
C1—S1 | 1.761 (4) | 1.761 (4) |
C1—S2 | 1.688 (4) | 1.678 (4) |
C1—N1 | 1.330 (5) | 1.342 (5) |
S1—Sn···S2 | 63.26 (3) | 63.42 (3) |
S1—Sn···C11 | 121.53 (10) | 111.30 (9) |
S1—Sn···C31 | 90.00 (11) | 92.68 (9) |
C11—Sn—C21 | 114.88 (15) | 119.27 (13) |
S2—Sn···C31 | 152.54 (11) | 155.43 (9) |
Summary of short interatomic contacts (Å) in (I) and (II) topContact | Distance | Symmetry operation |
(I) | | |
H2C···H7B | 1.98 | x, - 1 + y, z |
H2C···C7 | 2.67 | x, - 1 + y, z |
H23···C32 | 2.57 | - x, - 1 + y, - 1 + z |
H26···C33 | 2.70 | 1 - x, - 1 - y, - z |
(II) | | |
H2A···H7 | 2.26 | 2 - x, 1/2 + y, 3/2 + z |
H9···H23 | 2.29 | 1 + x, y, z |
H2A···C7 | 2.68 | 2 - x, 1/2 + y, 3/2 + z |
H2A···C8 | 2.74 | 2 - x, 1/2 + y, 3/2 + z |
H2B···C11 | 2.70 | 2 - x, - y, 2 - z |
H2B···C16 | 2.77 | 2 - x, - y, 2 - z |
H22···C35 | 2.69 | 1 - x, - y, 2 - z |
Percentage contributions of interatomic contacts to the Hirshfeld surface for (I) and (II) topContact | Percentage contribution | |
| (I) | (II) |
H···H | 66.1 | 57.8 |
C···H/H···C | 25.6 | 33.7 |
S···H/H···S | 7.6 | 7.6 |
N···H/H···N | 0.4 | 0.6 |
C···N/N···C | 0.2 | 0.0 |
S···N/N···S | 0.1 | 0.0 |
C···S/S···C | 0.0 | 0.3 |
Selected interatomic parameters (Å) for Ph3Sn(S2CNRR') topCompound | R | R' | Sn—Sshort | Sn—Slong | Δ(Sn—S) | Reference |
(III) | Et | Et | 2.429 (3) | 3.096 (3) | 0.67 | Hook et al. (1994) |
(IV)a | (CH2CH2)2C(H)CH2CH2 | (CH2CH2)2C(H)CH2 | 2.521 (3) | 2.919 (3) | 0.40 | Ali et al. (2014) |
| | | 2.4735 (10) | 2.9468 (10) | 0.47 | |
(V) | CH2Ph | CH2CH2Ph | 2.4885 (5) | 2.9120 (5) | 0.42 | Mohamad, Awang, Kamaludin, Jotani et al. (2016) |
(VI)b | CH2(3-pyridyl) | CH2(3-pyridyl) | 2.5165 (19) | 3.2209 (19) | 0.71 | Gupta et al. (2015) |
| | | 2.4685 (19) | 3.0397 (19) | 0.57 | |
Notes: (a) non-symmetric binuclear molecule; (b) two independent molecules in the asymmetric unit. |
Acknowledgements
We gratefully acknowledge the School of Chemical Sciences and Food Technology, Universiti Kebangsaan Malaysia, for providing the essential laboratory facilities. We would also like to thank the laboratory assistants of the Faculty of Science and Technology, Universiti Kebangsaan Malaysia, for technical support received. Intensity data were collected in the University of Malaya's crystallographic laboratory.
Funding information
This work was supported by the Fundamental Research Grant Scheme (FRGS/2/2013/SKK10/UKM/02/1) awarded by the Ministry of Education (MOE).
References
Addison, A. W., Rao, T. N., Reedijk, J., van Rijn, J. & Verschoor, G. C. (1984). J. Chem. Soc. Dalton Trans. pp. 1349–1356. CSD CrossRef Web of Science Google Scholar
Agilent (2015). CrysAlis PRO. Agilent Technologies Inc., Santa Clara, CA, USA. Google Scholar
Ali, S., Zia-ur-Rehman, Muneeb-ur-Rehman, Khan, I., Shah, S. N. A., Ali, R. F. Shaha, A., Badshah, A., Akbar, K. & Bélanger-Gariepy, F. (2014). J. Coord. Chem. 67, 3414–3430. Web of Science CSD CrossRef CAS Google Scholar
Altomare, A., Cascarano, G., Giacovazzo, C. & Guagliardi, A. (1993). J. Appl. Cryst. 26, 343–350. CrossRef Web of Science IUCr Journals Google Scholar
Brandenburg, K. (2006). DIAMOND. Crystal Impact GbR, Bonn, Germany. Google Scholar
Buntine, M. A., Hall, V. J., Kosovel, F. J. & Tiekink, E. R. T. (1998). J. Phys. Chem. A 102, 2472–2482. Web of Science CSD CrossRef CAS Google Scholar
Dance, I. & Scudder, M. (2009). CrystEngComm, 11, 2233–2247. Web of Science CrossRef CAS Google Scholar
Farrugia, L. J. (2012). J. Appl. Cryst. 45, 849–854. Web of Science CrossRef CAS IUCr Journals Google Scholar
Green, M., Prince, P., Gardener, M. & Steed, J. (2004). Adv. Mater. 16, 994–996. Web of Science CSD CrossRef CAS Google Scholar
Groom, C. R., Bruno, I. J., Lightfoot, M. P. & Ward, S. C. (2016). Acta Cryst. B72, 171–179. Web of Science CSD CrossRef IUCr Journals Google Scholar
Gupta, A. N., Kumar, V., Singh, V., Rajput, A., Prasad, L. B., Drew, M. G. B. & Singh, N. (2015). J. Organomet. Chem. 787, 65–72. Web of Science CSD CrossRef CAS Google Scholar
Heard, P. J. (2005). Prog. Inorg. Chem. 53, 1–69. Web of Science CrossRef CAS Google Scholar
Hogarth, G. (2012). Mini Rev. Med. Chem. 12, 1202–1215. Web of Science CrossRef CAS PubMed Google Scholar
Hook, J. M., Linahan, B. M., Taylor, R. L., Tiekink, E. R. T., van Gorkom, L. & Webster, L. K. (1994). Main Group Met. Chem. 17, 293–311. CrossRef CAS Google Scholar
Jotani, M. M., Tan, Y. S. & Tiekink, E. R. T. (2016). Z. Kristallogr. 231, 403–413. CAS Google Scholar
Lai, C. S. & Tiekink, E. R. T. (2007). Z. Kristallogr. 222, 532–538. Web of Science CSD CrossRef CAS Google Scholar
McKinnon, J. J., Jayatilaka, D. & Spackman, M. A. (2007). Chem. Commun. pp. 3814–3816. Web of Science CrossRef Google Scholar
Mohamad, R., Awang, N., Jotani, M. M. & Tiekink, E. R. T. (2016). Acta Cryst. E72, 1130–1137. Web of Science CSD CrossRef IUCr Journals Google Scholar
Mohamad, R., Awang, N. & Kamaludin, N. F. (2016). Res. J. Pharm. Biol. Chem. Sci. 7, 1920–1925. CAS Google Scholar
Mohamad, R., Awang, N., Kamaludin, N. F. & Abu Bakar, N. F. (2016). Res. J. Pharm. Biol. Chem. Sci. 7, 1269–1274. CAS Google Scholar
Mohamad, R., Awang, N., Kamaludin, N. F., Jotani, M. M. & Tiekink, E. R. T. (2016). Acta Cryst. E72, 1480–1487. Web of Science CSD CrossRef IUCr Journals Google Scholar
Mohamad, R., Awang, N., Kamaludin, N. F., Jotani, M. M. & Tiekink, E. R. T. (2017). Acta Cryst. E73, 260–265. Web of Science CSD CrossRef IUCr Journals Google Scholar
Mohamad, R., Awang, N., Kamaludin, N. F., Jotani, M. M. & Tiekink, E. R. T. (2018). Acta Cryst. E74, 302–308. Web of Science CSD CrossRef IUCr Journals Google Scholar
Monteiro, O. C., Nogueira, H. I. S., Trindade, T. & Motevalli, M. (2001). Chem. Mater. 13, 2103–2111. Web of Science CSD CrossRef CAS Google Scholar
Muthalib, A. F. A., Baba, I., Khaledi, H., Ali, H. M. & Tiekink, E. R. T. (2014). Z. Kristallogr. 229, 39–46. Google Scholar
Park, J.-H., Afzaal, M., Kemmler, M., O'Brien, P., Otway, D. J., Raftery, J. & Waters, J. (2003). J. Mater. Chem. 13, 1942–1949. Web of Science CSD CrossRef CAS Google Scholar
Ramasamy, K., Kuznetsov, V. L., Gopal, K., Malik, M. A., Raftery, J., Edwards, P. P. & O'Brien, P. (2013). Chem. Mater. 25, 266–276. Web of Science CSD CrossRef CAS Google Scholar
Segovia, N., Crovetto, G., Lardelli, P. & Espigares, M. (2002). J. Appl. Toxicol. 22, 353–357. Web of Science CrossRef CAS Google Scholar
Sheldrick, G. M. (2015). Acta Cryst. C71, 3–8. Web of Science CrossRef IUCr Journals Google Scholar
Spek, A. L. (2009). Acta Cryst. D65, 148–155. Web of Science CrossRef CAS IUCr Journals Google Scholar
Tiekink, E. R. T. (2008). Appl. Organomet. Chem. 22, 533–550. Web of Science CrossRef CAS Google Scholar
Tiekink, E. R. T., Hall, V. J. & Buntine, M. A. (1999). Z. Kristallogr. 214, 124–134. Web of Science CSD CrossRef CAS Google Scholar
Westrip, S. P. (2010). J. Appl. Cryst. 43, 920–925. Web of Science CrossRef CAS IUCr Journals Google Scholar
This is an open-access article distributed under the terms of the Creative Commons Attribution (CC-BY) Licence, which permits unrestricted use, distribution, and reproduction in any medium, provided the original authors and source are cited.
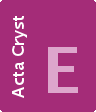 | CRYSTALLOGRAPHIC COMMUNICATIONS |
ISSN: 2056-9890
Open

access