1. Chemical context
Sulfur donor atoms bound to iron or nickel ions are commonly found in the active site of hydrogenase enzymes in nature. In [NiFe] hydrogenases, cysteine sulfurs are bound to the metal centers, and in [FeFe] hydrogenases, the amine moiety in the azadithiolate ligand bound to the iron centers is essential to the catalytic function (Lubitz et al., 2014
). Nickel(II) complexes with sulfur and nitrogen donor atoms are efficient precatalysts or real catalysts for the electro- and photoreduction of protons (Han et al., 2012
; Martin et al., 2015
; Luo et al., 2017
; Inoue et al., 2020
). It has been pointed out that the hemilabile pyridine ligand in [Ni(C5H4NS)3] is protonated in the photocatalytic hydrogen production (Han et al., 2012
). The pendant amines as a proton acceptor site are also important for developing efficient electrocatalysts for hydrogen production (Helm et al., 2011
; Stewart et al., 2013
). In this context, thiolate complexes with pendant amino groups are good candidates for the development of proton-reduction catalysts.
The nickel(II) complex [Ni(C11H16N3S)]Cl (Bouwman et al., 1999
) contains an N,N′,N′′,S-tetradentate Schiff base ligand with terminal thiolate and amine moieties. The terminal amino group that is bound to the Ni center is a potential proton-acceptor site. For instance, the Schiff base ligands derived from salicylaldehydes and 1-(2-aminoethyl)piperazine give square-planar and/or octahedral nickel(II) complexes, in which the terminal piperazinyl group binds to Ni in the bidentate chelate mode and the monodentate mode with protonation (Mukhopadhyay et al., 2003
). Furthermore the cationic complex [Ni(C11H16N3S)]Cl is water-soluble, which makes it possible to investigate its catalytic performance in aqueous media. In the electrocatalytic proton reduction, the electrochemical properties of the precatalysts are directly related to the formation of real catalysts. Therefore the tuning of the redox properties is also required in the ligand design.
In this work we synthesized two water-soluble N3S Schiff base nickel(II) complexes, [Ni(C12H18N3S)]Cl (1) and [Ni(C13H18N3S)]PF6 (2), in which the N,N′,N′′,S-tetradentate Schiff base ligands contain an additional N-methyl group and a terminal piperazine moiety, respectively. The electrochemical properties of these complexes were investigated by cyclic voltammetry in water, and compared with those of [Ni(C11H16N3S)]Cl (3) without N-substituents.
2. Structural commentary
The complex cations in 1 and 2 consist of an Ni2+ ion and a monoanionic N,N′,N′′,S-tetradentate ligand, giving a square-planar geometry. The asymmetric unit in 1 comprises the complex cation and a chloride anion, whereas in 2 a hexafluorophosphate anion and a dichloromethane molecule are incorporated into the crystal lattice.
Each complex cation contains a six-membered chelate ring with N and S donor atoms and two five-membered chelate rings with two N donor atoms (Fig. 1
and Fig. 2
). In the N,S-chelate, the N1—Ni1—S1 angles are 97.77 (5)° in 1 (Table 1
) and 98.90 (7)° in 2 (Table 2
), which are comparable to those in 3 [98.5 (2)°] and the tetraphenylborate salt [Ni(C11H16N3S)]B(C6H5)4 [3′; 95.8 (2)°]. The bond distances in the chelate rings are also comparable (Bouwman et al., 1999
; Goswami & Eichhorn, 1999
). The structural parameters of the central five-membered N,N-chelate rings of these complexes are similar to each other: the N1—Ni1—N2 angles are 86.53 (6)° in 1, 87.80 (10)° in 2, 86.1 (3)° in 3 (Bouwman et al., 1999
), and 87.8 (3)° in 3′ (Goswami & Eichhorn, 1999
). The bite angles of the N,N-chelate are also similar to those of the nickel(II) complexes with S,N,N,S-tetradentate ligands, which have two amine N or two imine N donor atoms besides two S atoms (Yamamura et al., 1993
). The structures of the central NCH2CH2N chelate are not significantly dependent on the terminal chelates of the tetradentate ligands. In the terminal N,N-chelate ring, the bite angle of the piperazine moiety is significantly restricted. The N2—Ni1—N3 angle of 2 [76.05 (10)°] is much smaller than those of 1 [86.16 (6)°], 3 [86.3 (3)°; Bouwman et al., 1999
], and 3′ [86.9 (3)°; Goswami & Eichhorn, 1999
], although the Ni—N distances match well with each other.
Ni1—N1 | 1.8587 (14) | Ni1—S1 | 2.1421 (5) | Ni1—N3 | 1.9268 (14) | S1—C1 | 1.7396 (17) | Ni1—N2 | 1.9345 (13) | N1—C7 | 1.289 (2) | | | | | N1—Ni1—N3 | 172.68 (6) | N1—Ni1—S1 | 97.77 (5) | N1—Ni1—N2 | 86.53 (6) | N3—Ni1—S1 | 89.53 (5) | N3—Ni1—N2 | 86.16 (6) | N2—Ni1—S1 | 175.49 (4) | | | | | N1—C8—C9A—N2 | 42.9 (2) | | | | |
Ni1—N1 | 1.843 (2) | Ni1—S1 | 2.1316 (10) | Ni1—N3 | 1.917 (3) | S1—C1 | 1.745 (3) | Ni1—N2 | 1.924 (2) | N1—C7 | 1.285 (3) | | | | | N1—Ni1—N3 | 162.95 (10) | N1—Ni1—S1 | 98.90 (7) | N1—Ni1—N2 | 87.80 (10) | N3—Ni1—S1 | 97.67 (8) | N3—Ni1—N2 | 76.05 (10) | N2—Ni1—S1 | 171.77 (7) | | | | | N1—C8—C9—N2 | 45.1 (3) | | | | |
| Figure 1 Perspective view of the complex cation of 1 with displacement ellipsoids at the 50% probability level. Hydrogen atoms of the minor occupancy component of the disordered region are omitted for clarity. |
| Figure 2 Perspective view of the complex cation of 2 with displacement ellipsoids at the 50% probability level. |
In complex 1, the methylene chains in the two N,N-chelate rings and the methyl group on the tertiary amine N atom are disordered over two sets of sites. These two models are enantiomers to each other. The conformation of the methylene chains is dependent on the configuration of the methyl group. The N,S-chelate ring in 1 does not show disorder, and the benzene ring and the NiN3S coordination plane are almost coplanar. The dihedral angle between the least-squares planes is 9.74 (8)°. The corresponding interplanar angle in 2 is 20.92 (12)°. Because there is no significant difference in the conformation of the central chelate ring between 1 [N1—C8—C9A—N2 = 42.9 (2)°] and 2 [N1—C8—C9—N2 = 45.1 (3)°], this bending is due to the rigid structure of the piperazine chelate, which fixes the direction of the methylene groups on the tertiary amine N atom.
3. Supramolecular features
The crystal structure of 1 shows hydrogen bonds between the terminal amine nitrogen atom in the complex cation and two chloride ions with the N3⋯Cl1 and N3⋯Cl1(−x +
, y −
, −z +
) distances of 3.2245 (17) and 3.1948 (17) Å, respectively (Table 3
), which are similar to that of 3 (Bouwman et al., 1999
). Each chloride ion bridges two complex cations through the hydrogen bonds. Thus in the crystal, the cations and anions pack together to form a zigzag hydrogen-bonded chain along the b-axis direction (Fig. 3
). The disorder found in the complex cation does not affect the chain structure. There are π–π interactions between the hydrogen-bonded chains through the planar N,S-chelate moieties including the benzene rings [centroid–centroid distances = 3.7378 (12) and 3.8965 (13) Å].
D—H⋯A | D—H | H⋯A | D⋯A | D—H⋯A | N3—HN3A⋯Cl1 | 0.93 (2) | 2.40 (2) | 3.2245 (17) | 148.7 (18) | N3—HN3B⋯Cl1i | 0.841 (19) | 2.41 (2) | 3.1948 (17) | 156.0 (17) | Symmetry code: (i) . | |
| Figure 3 Hydrogen-bond network of 1. Hydrogen atoms are omitted for clarity. Hydrogen bonds are shown as red dashed lines. [Symmetry codes: (i) −x + , y − , −z + ; (ii) −x + , y + , −z + ; (iii) x, y + 1, z; (iv) x, y − 1, z; (v) −x + 1, −y + 1, −z + 1; (vi) x + , −y + , z + ; (vii) x + , −y + , z + ; (viii) −x + 1, −y, −z + 1; (ix) −x + 1, −y + 2, −z + 1.] |
Several intermolecular C—H⋯π interactions exist in 2 between the methylene hydrogen atoms of the polyamine moiety and the π system of the benzene ring (Table 4
). The piperazine nitrogen atom N3 in the ligand forms a hydrogen bond to the hexafluorophosphate ion with the N3⋯F6(−x + 1, −y + 1, −z + 1) distance of 3.114 (3) Å (Table 4
). In addition, there are short contacts between the hexafluorophosphate ion and the methylene hydrogen atoms of the ligand [F6⋯H14B(x, y, z − 1) = 2.47 (4) Å].
D—H⋯A | D—H | H⋯A | D⋯A | D—H⋯A | N3—H3N⋯F6i | 0.80 (3) | 2.50 (3) | 3.114 (3) | 135 (3) | C10—H10A⋯C4ii | 0.96 (3) | 2.85 (3) | 3..695 (5) | 147 (2) | C8—H8A⋯Cg6ii | 0.98 (3) | 2.84 (3) | 3.778 (4) | 160 (2) | Symmetry codes: (i) ; (ii) . | |
4. Database survey
The two N,N′,N′′,S-tetradentate Schiff base ligands studied here have not been reported so far for other transition-metal ions. A similar Schiff base structure that contains benzenethiolate and polyamines is found in a trinuclear nickel(II) complex with a C3-symmetric ligand based on a 1,3,5-trimercaptobenzene backbone (Feldscher et al., 2014
). An analogous mononuclear nickel(II) complex that has a phenol O atom instead of the thiol S atom in 2 shows a piperazine bite angle of 76.65 (8)° (Mukhopadhyay et al., 2003
), which is comparable to that of 2.
5. Spectroscopic features
The solution structures of 1 and 2 were characterized by 1H and 1H–1H COSY NMR spectroscopy in methanol-d4. The 1H NMR spectrum of 1 exhibits an azomethine proton at 8.16 ppm and four aromatic protons in the range 7.07–7.65 ppm (Fig. 4
). In the aliphatic region, eight multiplet signals and a singlet signal are due to methylene and methyl groups, respectively. The COSY spectrum of 1 shows cross peaks between the azomethine proton and the two methylene protons at 4.01 and 4.35 ppm; thus, they were attributed to the CH2 group adjacent to the C=N group. Similar spectroscopic features appear for 2 in the aromatic region, whereas six sets of signals due to methylene protons are observed in the aliphatic region. The two sharp signals at 2.83 and 4.31 ppm for 2 were attributed to the central N,N-chelate moiety, and the latter is assigned to the CH2 group adjacent to C=N on the basis of the COSY correlation. This observation is consistent with the fast conformational change of the central chelate ring in 2. Furthermore, the similar signal pattern for the terminal methylene protons at 3.96 and 4.21 ppm suggests a boat conformation of the piperazine moiety that binds to Ni in the bidentate chelate mode.
| Figure 4 1H NMR spectra of (a) 1 (400 MHz) and (b) 2 (300 MHz) in CD3OD. |
6. Electrochemical Properties
The redox behavior of the N,N′,N′′,S-tetradentate Schiff base nickel(II) complexes 1, 2, and 3 was investigated by cyclic voltammetry. Measurements were performed in 5 × 10−4 M (1 M = 1 mol dm−3) aqueous solution containing KNO3 (0.1 M) at a scan rate of 0.1 V s−1. The working electrode was a glassy carbon disk electrode with a diameter of 3 mm, the auxiliary electrode was a platinum wire, and the reference electrode was Ag/AgCl/saturated KCl. All complexes exhibit irreversible reduction and oxidation processes (Fig. 5
). In the reduction process, the cathodic wave appeared at −1.31 V for 1, −1.19 V for 2, and −1.34 V for 3. The anodic peaks in the reverse scan (–0.52 V for 1; −0.70, −0.40 V for 2, and −0.48 V for 3) suggest the adsorption of the reduced species. In the oxidation process, the anodic wave appeared at 0.73 V for 1, 0.79 V for 2, and 0.68 V for 3. In both processes, the redox potentials of 1 are slightly shifted to more positive values than those of 3, which suggests that the electronic and steric effects of the methyl group on the central N atom are not so significant. The voltammogram of 2 shows further positive shifts, and the shift in the reduction process is more pronounced. This is probably related to the smaller bite angle of the terminal piperazine chelate, which reduces the electron-donating ability of the Schiff base ligand toward the nickel center.
The proton-reduction abilities of complexes 1 and 2 were compared in a buffer solution of pH 4.6 (0.1 M acetic acid/sodium acetate). A catalytic current was observed during the reduction process, giving a peak at −1.28 V for 1 and −1.23 V for 2 (Fig. 6
). This suggests that the reduced species of the nickel(II) complex is catalytically active for proton reduction. The reduction potential for 2 is more positive than that for 1, and thus the piperazinyl arm in 2 is effective in reducing the overpotential for proton reduction.
| Figure 6 Cyclic voltammograms of complexes 1 (0.5 mM, red dotted line), 2 (0.5 mM, blue solid line), and blank solution (black dashed line) in 0.1 M acetate buffer at pH 4.6 containing 0.10 M KNO3: scan rate, 0.1 V s−1; working electrode, glassy carbon; auxiliary electrode, platinum wire; reference electrode, Ag/AgCl/saturated KCl. |
7. Synthesis and crystallization
General Procedures. NMR spectra were recorded on a Bruker AVANCE 300 or a JEOL EX-400 spectrometer at room temperature. Cyclic voltammetric measurements were performed at room temperature with an ALS/DY2325 voltammetric analyzer (Bioanalytical System Ins.) under N2. Elemental analyses were performed by the Analytical Research Service Center at Osaka City University or A Rabbit Science Co., Ltd.
[Ni(C12H18N3S)]Cl (1). A solution of 2,2′-diamino-N-methyldiethylamine (128 µL, 1.0 mmol) and 2-(t-butylthio)benzaldehyde (194 mg, 1.0 mmol) in ethanol (10 mL) was refluxed under a nitrogen atmosphere for 1 h to afford a pale-yellow solution. After cooling to room temperature, NiCl2·6H2O (238 mg, 1.0 mmol) was added, and the resulting green suspension was refluxed under a nitrogen atmosphere for 6 h, during which time the color of the solution turned red. The reaction mixture was cooled to room temperature and filtered. The red filtrate was left for two weeks to give red crystals of 1 (41 mg, 12%). 1H NMR (400 MHz, CD3OD): δ 2.65 (dd, J = 12.1, 4.9 Hz, 1H, C=NCH2CH2N), 2.67 (dd, J = 12.2, 4.5 Hz, 1H, NCH2CH2NH2), 2.79 (dd, J = 13.3, 5.9 Hz, 1H, NCH2CH2NH2), 2.92 (dd, J = 13.3, 4.5 Hz, 1H, NCH2CH2NH2), 2.95 (s, 3H, NMe), 3.43 (td, J = 12.8, 5.9 Hz, 1H, NCH2CH2NH2), 3.54 (td, J = 12.8, 6.1 Hz, 1H, C=NCH2CH2N), 4.01 (dd, J = 15.1, 6.1 Hz, 1H, C=NCH2CH2N), 4.35 (dddd, J = 15.1, 13.5, 4.9, 1.7 Hz, 1H, C=NCH2CH2N), 7.07 (ddd, J = 7.8, 7.2, 1.1 Hz, 1H, Ar) 7.25 (ddd, J = 8.0, 7.2, 1.4 Hz 1H, Ar), 7.49 (dd, J = 8.0, 1.3 Hz, 1H, Ar), 7.65 (d, J = 8.1 Hz, 1H, Ar), 8.16 (s, 1H, N=CH). Analysis calculated for C12H18ClN3NiS·0.25H2O: C, 43.02; H, 5.57; N, 12.54. Found: C, 42.94; H, 5.28; N, 12.48.
[Ni(C13H18N3S)]PF6 (2). To a solution of N-(2-aminoethyl)piperazine (261 mg, 2.0 mmol) and 2-(t-butylthio)benzaldehyde (490 mg, 2.1 mmol) in ethanol (20 mL) was added NiCl2·6H2O (490 mg, 2.1 mmol). The resulting suspension was refluxed under a nitrogen atmosphere for 8 h, during which time the color of the solution turned orange and a yellow–green precipitate formed. The reaction mixture was filtered, and an ethanol solution (5 mL) of ammonium hexafluorophosphate (334 mg, 2.1 mmol) was added to the filtrate. The resulting orange precipitate was collected by filtration and dried under reduced pressure to give an orange powder of 2 (353 mg, 38%). Suitable crystals for X-ray diffraction analysis were grown from a dichloromethane solution by layering with diethyl ether. 1H NMR (300 MHz, CD3OD): δ 2.76–2.94 (m, 4H, N(CH2CH2)2NH), 2.83 (t, J = 6.5 Hz, 2H, C=NCH2CH2N), 3.91–4.03 (m, 2H, N(CH2CH2)2NH), 4.16–4.27 (m, 2H, N(CH2CH2)2NH), 4.31 (td, J = 6.5, 1.2 Hz, 2H, C=NCH2CH2N), 7.10 (td, J = 7.9, 1.2 Hz, 1H, Ar), 7.28 (td, J = 8.2, 1.5 Hz, 1H, Ar), 7.52 (dd, J = 7.9, 1.5 Hz, 1H, Ar), 7.74 (d, J = 8.2 Hz, 1H, Ar), 8.16 (s, 1H, N=CH). Analysis calculated for C13H18F6N3NiPS·0.75CH2Cl2: C, 32.02; H, 3.81; N, 8.15. Found: C, 32.11; H, 3.93; N, 8.14.
8. Refinement
Crystal data, data collection and structure refinement details are summarized in Table 5
. All non-hydrogen atoms were refined anisotropically. A methyl group and two methylene groups bound to the central N atom of two N,N-chelating moieties in 1 were modeled as disordered over two positions each, and the occupancy factors refined to 0.864 (3) and 0.136 (3). Hydrogen atoms on the disordered C atoms and the adjacent C atoms that belong to the minor site were placed in calculated positions with C—H(methyl) = 0.98 Å and C—H(methylene) = 0.99 Å and refined using a riding model with Uiso(H) = 1.5Ueq(C) and 1.2Ueq(C), respectively. Other H atoms were found in a difference-Fourier map and freely refined.
| 1 | 2 | Crystal data | Chemical formula | [Ni(C12H18N3S)]Cl | [Ni(C13H18N3S)]PF6·CH2Cl2 | Mr | 330.51 | 536.97 | Crystal system, space group | Monoclinic, P21/n | Triclinic, P![[\overline{1}]](teximages/dj2045fi7.svg) | Temperature (K) | 153 | 153 | a, b, c (Å) | 7.8601 (14), 9.8884 (17), 18.190 (3) | 8.725 (3), 10.507 (4), 11.316 (4) | α, β, γ (°) | 90, 98.677 (3), 90 | 98.065 (4), 101.274 (6), 96.150 (5) | V (Å3) | 1397.6 (4) | 997.6 (6) | Z | 4 | 2 | Radiation type | Mo Kα | Mo Kα | μ (mm−1) | 1.71 | 1.49 | Crystal size (mm) | 0.11 × 0.06 × 0.04 | 0.17 × 0.11 × 0.08 | | Data collection | Diffractometer | Rigaku AFC11 with Saturn 724+ CCD | Rigaku AFC11 with Saturn 724+ CCD | Absorption correction | Multi-scan (REQAB; Rigaku, 1998 ) | Multi-scan (REQAB; Rigaku, 1998 ) | Tmin, Tmax | 0.904, 1.000 | 0.828, 1.000 | No. of measured, independent and observed [I > 2σ(I)] reflections | 11199, 3129, 2665 | 8155, 4392, 3240 | Rint | 0.024 | 0.041 | (sin θ/λ)max (Å−1) | 0.649 | 0.649 | | Refinement | R[F2 > 2σ(F2)], wR(F2), S | 0.024, 0.057, 1.05 | 0.037, 0.087, 0.93 | No. of reflections | 3129 | 4392 | No. of parameters | 263 | 333 | H-atom treatment | H atoms treated by a mixture of independent and constrained refinement | All H-atom parameters refined | Δρmax, Δρmin (e Å−3) | 0.43, −0.24 | 0.79, −0.52 | Computer programs: CrystalClear (Rigaku, 2008 ), SIR97 (Altomare et al., 1999 ), SHELXL2013 (Sheldrick, 2015 ), ORTEP-3 for Windows and WinGX (Farrugia, 2012 ), and Mercury (Macrae et al., 2020 ). | |
Supporting information
For both structures, data collection: CrystalClear (Rigaku, 2008); cell refinement: CrystalClear (Rigaku, 2008); data reduction: CrystalClear (Rigaku, 2008); program(s) used to solve structure: SIR97 (Altomare et al., 1999); program(s) used to refine structure: SHELXL2013 (Sheldrick, 2015); molecular graphics: ORTEP-3 for Windows (Farrugia, 2012), Mercury (Macrae et al., 2020); software used to prepare material for publication: WinGX (Farrugia, 2012).
{2-[({2-[(2-Aminoethyl-
κN)(methyl)amino-
κN]ethyl}imino-
κN)methyl]benzenethiolato-
κS}nickel(II) chloride (1)
top Crystal data top [Ni(C12H18N3S)]Cl | F(000) = 688 |
Mr = 330.51 | Dx = 1.571 Mg m−3 |
Monoclinic, P21/n | Mo Kα radiation, λ = 0.71075 Å |
a = 7.8601 (14) Å | Cell parameters from 4130 reflections |
b = 9.8884 (17) Å | θ = 3.1–27.5° |
c = 18.190 (3) Å | µ = 1.71 mm−1 |
β = 98.677 (3)° | T = 153 K |
V = 1397.6 (4) Å3 | Prism, red |
Z = 4 | 0.11 × 0.06 × 0.04 mm |
Data collection top Rigaku AFC11 with Saturn 724+ CCD diffractometer | 3129 independent reflections |
Radiation source: Rotating Anode | 2665 reflections with I > 2σ(I) |
Detector resolution: 28.5714 pixels mm-1 | Rint = 0.024 |
ω scans | θmax = 27.5°, θmin = 3.1° |
Absorption correction: multi-scan (REQAB; Rigaku, 1998) | h = −10→10 |
Tmin = 0.904, Tmax = 1.000 | k = −12→11 |
11199 measured reflections | l = −23→22 |
Refinement top Refinement on F2 | Primary atom site location: structure-invariant direct methods |
Least-squares matrix: full | Secondary atom site location: difference Fourier map |
R[F2 > 2σ(F2)] = 0.024 | Hydrogen site location: mixed |
wR(F2) = 0.057 | H atoms treated by a mixture of independent and constrained refinement |
S = 1.05 | w = 1/[σ2(Fo2) + (0.0319P)2 + 0.1838P] where P = (Fo2 + 2Fc2)/3 |
3129 reflections | (Δ/σ)max = 0.001 |
263 parameters | Δρmax = 0.43 e Å−3 |
0 restraints | Δρmin = −0.24 e Å−3 |
Special details top Geometry. All esds (except the esd in the dihedral angle between two l.s. planes) are estimated using the full covariance matrix. The cell esds are taken into account individually in the estimation of esds in distances, angles and torsion angles; correlations between esds in cell parameters are only used when they are defined by crystal symmetry. An approximate (isotropic) treatment of cell esds is used for estimating esds involving l.s. planes. |
Refinement. A methyl group and two methylene groups bound to N2 were modeled as disordered over two positions each, and the occupancy factors were refined to 0.864 (3) and 0.136 (3). |
Fractional atomic coordinates and isotropic or equivalent isotropic displacement parameters (Å2) top | x | y | z | Uiso*/Ueq | Occ. (<1) |
Ni1 | 0.48400 (3) | 0.13970 (2) | 0.38534 (2) | 0.01822 (7) | |
Cl1 | 0.40893 (5) | 0.34256 (4) | 0.18937 (2) | 0.02646 (10) | |
S1 | 0.26456 (5) | 0.23714 (4) | 0.41873 (2) | 0.02246 (10) | |
N1 | 0.64016 (17) | 0.17644 (15) | 0.47031 (8) | 0.0235 (3) | |
N2 | 0.66981 (17) | 0.04423 (14) | 0.34932 (8) | 0.0215 (3) | |
N3 | 0.34538 (19) | 0.08851 (16) | 0.29300 (8) | 0.0225 (3) | |
C1 | 0.3229 (2) | 0.33075 (16) | 0.49958 (9) | 0.0217 (3) | |
C2 | 0.1926 (2) | 0.4054 (2) | 0.52601 (10) | 0.0288 (4) | |
C3 | 0.2272 (3) | 0.4853 (2) | 0.58865 (11) | 0.0329 (4) | |
C4 | 0.3922 (3) | 0.4947 (2) | 0.62816 (10) | 0.0330 (4) | |
C5 | 0.5199 (2) | 0.4198 (2) | 0.60486 (10) | 0.0293 (4) | |
C6 | 0.4898 (2) | 0.33615 (17) | 0.54095 (9) | 0.0236 (4) | |
C7 | 0.6332 (2) | 0.25697 (19) | 0.52548 (9) | 0.0270 (4) | |
C8 | 0.8076 (2) | 0.1028 (2) | 0.47227 (11) | 0.0344 (5) | |
C9A | 0.7758 (3) | −0.0122 (2) | 0.41595 (12) | 0.0309 (5) | 0.864 (3) |
C9B | 0.8355 (14) | 0.1034 (12) | 0.3869 (7) | 0.019 (3) | 0.136 (3) |
C10A | 0.5894 (3) | −0.0624 (2) | 0.29769 (13) | 0.0287 (5) | 0.864 (3) |
C11 | 0.4437 (2) | 0.0035 (2) | 0.24619 (10) | 0.0279 (4) | |
C10B | 0.6424 (15) | 0.0424 (13) | 0.2701 (7) | 0.022 (3) | 0.136 (3) |
C12A | 0.7731 (3) | 0.1392 (2) | 0.30855 (12) | 0.0277 (5) | 0.864 (3) |
C12B | 0.6622 (19) | −0.1036 (12) | 0.3796 (8) | 0.026 (3) | 0.136 (3) |
HN3A | 0.321 (3) | 0.170 (2) | 0.2682 (12) | 0.041 (6)* | |
HN3B | 0.259 (2) | 0.044 (2) | 0.3013 (10) | 0.025 (5)* | |
H2 | 0.077 (2) | 0.3969 (18) | 0.5015 (10) | 0.021 (5)* | |
H3 | 0.140 (3) | 0.530 (2) | 0.6037 (11) | 0.035 (6)* | |
H4 | 0.415 (3) | 0.551 (2) | 0.6699 (11) | 0.038 (6)* | |
H5 | 0.633 (3) | 0.417 (2) | 0.6294 (11) | 0.033 (5)* | |
H7 | 0.733 (2) | 0.2637 (19) | 0.5632 (10) | 0.028 (5)* | |
H8A | 0.895 (3) | 0.165 (2) | 0.4615 (12) | 0.022 (6)* | 0.864 (3) |
H8B | 0.840 (3) | 0.069 (2) | 0.5207 (12) | 0.027 (6)* | 0.864 (3) |
H8C | 0.7997 | 0.0094 | 0.4910 | 0.041* | 0.136 (3) |
H8D | 0.9022 | 0.1507 | 0.5039 | 0.041* | 0.136 (3) |
H9A | 0.887 (3) | −0.053 (3) | 0.4044 (13) | 0.041 (7)* | 0.864 (3) |
H9B | 0.698 (4) | −0.092 (3) | 0.4307 (15) | 0.045 (7)* | 0.864 (3) |
H9C | 0.8526 | 0.1963 | 0.3691 | 0.023* | 0.136 (3) |
H9D | 0.9350 | 0.0467 | 0.3791 | 0.023* | 0.136 (3) |
H10A | 0.675 (3) | −0.105 (2) | 0.2715 (13) | 0.032 (6)* | 0.864 (3) |
H10B | 0.547 (3) | −0.133 (2) | 0.3318 (14) | 0.030 (6)* | 0.864 (3) |
H10C | 0.7177 | −0.0255 | 0.2512 | 0.027* | 0.136 (3) |
H10D | 0.6670 | 0.1323 | 0.2501 | 0.027* | 0.136 (3) |
H11A | 0.485 (3) | 0.063 (2) | 0.2104 (12) | 0.022 (5)* | 0.864 (3) |
H11B | 0.371 (3) | −0.063 (2) | 0.2180 (12) | 0.024 (5)* | 0.864 (3) |
H11C | 0.4066 | 0.0228 | 0.1928 | 0.033* | 0.136 (3) |
H11D | 0.4251 | −0.0937 | 0.2552 | 0.033* | 0.136 (3) |
H12A | 0.818 (3) | 0.216 (2) | 0.3439 (13) | 0.036 (6)* | 0.864 (3) |
H12B | 0.696 (3) | 0.181 (2) | 0.2665 (13) | 0.029 (6)* | 0.864 (3) |
H12C | 0.868 (3) | 0.089 (2) | 0.2898 (13) | 0.037 (7)* | 0.864 (3) |
H12D | 0.6808 | −0.1020 | 0.4341 | 0.039* | 0.136 (3) |
H12E | 0.7519 | −0.1583 | 0.3620 | 0.039* | 0.136 (3) |
H12F | 0.5491 | −0.1428 | 0.3616 | 0.039* | 0.136 (3) |
Atomic displacement parameters (Å2) top | U11 | U22 | U33 | U12 | U13 | U23 |
Ni1 | 0.01721 (11) | 0.01974 (11) | 0.01704 (11) | 0.00138 (8) | 0.00044 (8) | 0.00006 (8) |
Cl1 | 0.0282 (2) | 0.0263 (2) | 0.0242 (2) | −0.00019 (16) | 0.00157 (17) | 0.00158 (16) |
S1 | 0.01838 (19) | 0.0278 (2) | 0.0201 (2) | 0.00215 (16) | −0.00091 (15) | −0.00525 (17) |
N1 | 0.0179 (7) | 0.0315 (8) | 0.0199 (7) | 0.0033 (6) | −0.0013 (6) | 0.0028 (6) |
N2 | 0.0220 (7) | 0.0199 (7) | 0.0227 (7) | 0.0028 (6) | 0.0034 (6) | 0.0028 (6) |
N3 | 0.0236 (8) | 0.0236 (7) | 0.0200 (7) | −0.0003 (6) | 0.0018 (6) | −0.0021 (6) |
C1 | 0.0253 (8) | 0.0226 (8) | 0.0168 (8) | −0.0018 (7) | 0.0020 (7) | −0.0004 (6) |
C2 | 0.0265 (9) | 0.0310 (9) | 0.0288 (10) | 0.0024 (7) | 0.0033 (8) | −0.0053 (8) |
C3 | 0.0399 (11) | 0.0317 (10) | 0.0286 (10) | 0.0046 (9) | 0.0103 (9) | −0.0052 (8) |
C4 | 0.0494 (12) | 0.0298 (9) | 0.0196 (9) | −0.0044 (9) | 0.0048 (8) | −0.0054 (8) |
C5 | 0.0321 (10) | 0.0354 (10) | 0.0185 (9) | −0.0071 (8) | −0.0019 (8) | −0.0017 (7) |
C6 | 0.0256 (8) | 0.0280 (9) | 0.0163 (8) | −0.0027 (7) | 0.0008 (7) | 0.0010 (7) |
C7 | 0.0229 (8) | 0.0372 (10) | 0.0186 (8) | −0.0014 (8) | −0.0041 (7) | 0.0007 (7) |
C8 | 0.0221 (9) | 0.0531 (13) | 0.0259 (10) | 0.0116 (9) | −0.0027 (8) | 0.0009 (9) |
C9A | 0.0289 (11) | 0.0346 (12) | 0.0286 (12) | 0.0113 (10) | 0.0026 (9) | 0.0095 (9) |
C9B | 0.011 (5) | 0.022 (6) | 0.025 (6) | 0.005 (4) | 0.004 (5) | −0.005 (5) |
C10A | 0.0343 (12) | 0.0205 (10) | 0.0317 (12) | 0.0030 (9) | 0.0066 (9) | −0.0043 (9) |
C10B | 0.020 (6) | 0.024 (6) | 0.025 (6) | −0.001 (5) | 0.009 (5) | 0.002 (5) |
C11 | 0.0296 (9) | 0.0296 (9) | 0.0242 (9) | 0.0016 (8) | 0.0034 (8) | −0.0069 (8) |
C12A | 0.0256 (11) | 0.0299 (11) | 0.0286 (11) | −0.0004 (9) | 0.0080 (9) | 0.0034 (9) |
C12B | 0.037 (7) | 0.016 (6) | 0.025 (7) | −0.009 (5) | 0.009 (6) | −0.003 (5) |
Geometric parameters (Å, º) top Ni1—N1 | 1.8587 (14) | C7—H7 | 0.96 (2) |
Ni1—N3 | 1.9268 (14) | C8—C9A | 1.526 (3) |
Ni1—N2 | 1.9345 (13) | C8—C9B | 1.601 (12) |
Ni1—S1 | 2.1421 (5) | C8—H8C | 0.9900 |
S1—C1 | 1.7396 (17) | C8—H8D | 0.9900 |
N1—C7 | 1.289 (2) | C8—H8A | 0.96 (2) |
N1—C8 | 1.499 (2) | C8—H8B | 0.94 (2) |
N2—C10B | 1.425 (12) | C11—C10A | 1.513 (3) |
N2—C9A | 1.473 (2) | C11—C10B | 1.604 (12) |
N2—C10A | 1.488 (2) | C11—H11C | 0.9900 |
N2—C9B | 1.496 (12) | C11—H11D | 0.9900 |
N2—C12A | 1.507 (2) | C11—H11A | 0.97 (2) |
N2—C12B | 1.566 (12) | C11—H11B | 0.96 (2) |
N3—C11 | 1.492 (2) | C9A—H9A | 1.02 (2) |
N3—HN3A | 0.93 (2) | C9A—H9B | 1.06 (3) |
N3—HN3B | 0.841 (19) | C10A—H10A | 0.97 (2) |
C1—C2 | 1.404 (2) | C10A—H10B | 1.02 (2) |
C1—C6 | 1.411 (2) | C12A—H12A | 1.02 (3) |
C2—C3 | 1.380 (3) | C12A—H12B | 0.99 (2) |
C2—H2 | 0.953 (19) | C12A—H12C | 1.00 (2) |
C3—C4 | 1.388 (3) | C9B—H9C | 0.9900 |
C3—H3 | 0.89 (2) | C9B—H9D | 0.9900 |
C4—C5 | 1.365 (3) | C10B—H10C | 0.9900 |
C4—H4 | 0.94 (2) | C10B—H10D | 0.9900 |
C5—C6 | 1.417 (2) | C12B—H12D | 0.9800 |
C5—H5 | 0.93 (2) | C12B—H12E | 0.9800 |
C6—C7 | 1.435 (2) | C12B—H12F | 0.9800 |
| | | |
N1—Ni1—N3 | 172.68 (6) | H8C—C8—H8D | 109.1 |
N1—Ni1—N2 | 86.53 (6) | N1—C8—H8A | 109.7 (13) |
N3—Ni1—N2 | 86.16 (6) | C9A—C8—H8A | 112.8 (13) |
N1—Ni1—S1 | 97.77 (5) | N1—C8—H8B | 107.9 (13) |
N3—Ni1—S1 | 89.53 (5) | C9A—C8—H8B | 110.8 (14) |
N2—Ni1—S1 | 175.49 (4) | H8A—C8—H8B | 108.6 (18) |
C1—S1—Ni1 | 111.00 (6) | N3—C11—C10A | 107.33 (15) |
C7—N1—C8 | 114.95 (14) | N3—C11—C10B | 106.1 (5) |
C7—N1—Ni1 | 131.91 (12) | N3—C11—H11C | 110.5 |
C8—N1—Ni1 | 113.07 (11) | C10B—C11—H11C | 110.5 |
C9A—N2—C10A | 112.64 (15) | N3—C11—H11D | 110.5 |
C10B—N2—C9B | 117.1 (7) | C10B—C11—H11D | 110.5 |
C9A—N2—C12A | 111.16 (15) | H11C—C11—H11D | 108.7 |
C10A—N2—C12A | 109.86 (15) | N3—C11—H11A | 107.3 (12) |
C10B—N2—C12B | 109.6 (7) | C10A—C11—H11A | 112.3 (12) |
C9B—N2—C12B | 106.3 (7) | N3—C11—H11B | 111.8 (12) |
C10B—N2—Ni1 | 110.0 (5) | C10A—C11—H11B | 111.6 (13) |
C9A—N2—Ni1 | 105.56 (11) | H11A—C11—H11B | 106.5 (17) |
C10A—N2—Ni1 | 106.80 (12) | N2—C9A—C8 | 106.59 (16) |
C9B—N2—Ni1 | 107.7 (4) | N2—C9A—H9A | 112.3 (13) |
C12A—N2—Ni1 | 110.66 (12) | C8—C9A—H9A | 112.1 (14) |
C12B—N2—Ni1 | 105.4 (5) | N2—C9A—H9B | 102.4 (15) |
C11—N3—Ni1 | 111.83 (11) | C8—C9A—H9B | 115.4 (14) |
C11—N3—HN3A | 107.1 (13) | H9A—C9A—H9B | 108 (2) |
Ni1—N3—HN3A | 104.2 (14) | N2—C10A—C11 | 107.20 (15) |
C11—N3—HN3B | 107.7 (13) | N2—C10A—H10A | 110.9 (14) |
Ni1—N3—HN3B | 110.2 (13) | C11—C10A—H10A | 113.2 (14) |
HN3A—N3—HN3B | 115.9 (19) | N2—C10A—H10B | 104.3 (13) |
C2—C1—C6 | 117.68 (15) | C11—C10A—H10B | 112.4 (14) |
C2—C1—S1 | 117.14 (13) | H10A—C10A—H10B | 108.4 (18) |
C6—C1—S1 | 125.17 (13) | N2—C12A—H12A | 108.2 (13) |
C3—C2—C1 | 121.38 (18) | N2—C12A—H12B | 109.1 (13) |
C3—C2—H2 | 119.5 (11) | H12A—C12A—H12B | 106.5 (19) |
C1—C2—H2 | 119.1 (11) | N2—C12A—H12C | 110.2 (14) |
C2—C3—C4 | 121.23 (18) | H12A—C12A—H12C | 112.4 (19) |
C2—C3—H3 | 118.2 (13) | H12B—C12A—H12C | 110.2 (18) |
C4—C3—H3 | 120.6 (13) | N2—C9B—C8 | 101.8 (7) |
C5—C4—C3 | 118.43 (17) | N2—C9B—H9C | 111.4 |
C5—C4—H4 | 120.9 (13) | C8—C9B—H9C | 111.4 |
C3—C4—H4 | 120.7 (13) | N2—C9B—H9D | 111.4 |
C4—C5—C6 | 122.11 (17) | C8—C9B—H9D | 111.4 |
C4—C5—H5 | 124.1 (13) | H9C—C9B—H9D | 109.3 |
C6—C5—H5 | 113.8 (12) | N2—C10B—C11 | 105.6 (7) |
C1—C6—C5 | 119.10 (16) | N2—C10B—H10C | 110.6 |
C1—C6—C7 | 124.70 (15) | C11—C10B—H10C | 110.6 |
C5—C6—C7 | 116.12 (15) | N2—C10B—H10D | 110.6 |
N1—C7—C6 | 128.09 (16) | C11—C10B—H10D | 110.6 |
N1—C7—H7 | 118.1 (11) | H10C—C10B—H10D | 108.7 |
C6—C7—H7 | 113.8 (11) | N2—C12B—H12D | 109.5 |
N1—C8—C9A | 106.96 (15) | N2—C12B—H12E | 109.5 |
N1—C8—C9B | 102.9 (4) | H12D—C12B—H12E | 109.5 |
N1—C8—H8C | 111.2 | N2—C12B—H12F | 109.5 |
C9B—C8—H8C | 111.2 | H12D—C12B—H12F | 109.5 |
N1—C8—H8D | 111.2 | H12E—C12B—H12F | 109.5 |
C9B—C8—H8D | 111.2 | | |
| | | |
N2—Ni1—N1—C7 | 168.82 (17) | C12A—N2—C9A—C8 | 71.6 (2) |
S1—Ni1—N1—C7 | −12.54 (17) | C12B—N2—C9A—C8 | −146.3 (7) |
N2—Ni1—N1—C8 | −7.83 (13) | Ni1—N2—C9A—C8 | −48.46 (18) |
S1—Ni1—N1—C8 | 170.81 (12) | N1—C8—C9A—N2 | 42.9 (2) |
Ni1—S1—C1—C2 | 177.10 (12) | C9B—C8—C9A—N2 | −50.5 (5) |
Ni1—S1—C1—C6 | −3.70 (17) | C10B—N2—C10A—C11 | −55.6 (6) |
C6—C1—C2—C3 | 2.3 (3) | C9A—N2—C10A—C11 | 162.30 (16) |
S1—C1—C2—C3 | −178.40 (15) | C9B—N2—C10A—C11 | −137.0 (9) |
C1—C2—C3—C4 | −0.2 (3) | C12A—N2—C10A—C11 | −73.2 (2) |
C2—C3—C4—C5 | −1.8 (3) | C12B—N2—C10A—C11 | 145.6 (6) |
C3—C4—C5—C6 | 1.6 (3) | Ni1—N2—C10A—C11 | 46.86 (18) |
C2—C1—C6—C5 | −2.5 (3) | N3—C11—C10A—N2 | −45.4 (2) |
S1—C1—C6—C5 | 178.33 (13) | C10B—C11—C10A—N2 | 50.7 (6) |
C2—C1—C6—C7 | 174.18 (17) | C10B—N2—C9B—C8 | 173.6 (7) |
S1—C1—C6—C7 | −5.0 (3) | C9A—N2—C9B—C8 | −47.8 (4) |
C4—C5—C6—C1 | 0.5 (3) | C10A—N2—C9B—C8 | −127.0 (6) |
C4—C5—C6—C7 | −176.40 (18) | C12A—N2—C9B—C8 | 153.0 (8) |
C8—N1—C7—C6 | −177.03 (18) | C12B—N2—C9B—C8 | −63.5 (8) |
Ni1—N1—C7—C6 | 6.4 (3) | Ni1—N2—C9B—C8 | 49.1 (6) |
C1—C6—C7—N1 | 5.2 (3) | N1—C8—C9B—N2 | −53.5 (6) |
C5—C6—C7—N1 | −178.09 (18) | C9A—C8—C9B—N2 | 48.0 (4) |
C7—N1—C8—C9A | 165.20 (17) | C9A—N2—C10B—C11 | 124.5 (6) |
Ni1—N1—C8—C9A | −17.6 (2) | C10A—N2—C10B—C11 | 50.5 (5) |
C7—N1—C8—C9B | −141.0 (5) | C9B—N2—C10B—C11 | −168.6 (6) |
Ni1—N1—C8—C9B | 36.2 (5) | C12A—N2—C10B—C11 | −148.4 (9) |
Ni1—N3—C11—C10A | 23.43 (19) | C12B—N2—C10B—C11 | 70.2 (9) |
Ni1—N3—C11—C10B | −26.0 (5) | Ni1—N2—C10B—C11 | −45.2 (8) |
C10B—N2—C9A—C8 | 141.6 (9) | N3—C11—C10B—N2 | 45.7 (8) |
C10A—N2—C9A—C8 | −164.64 (17) | C10A—C11—C10B—N2 | −53.3 (5) |
C9B—N2—C9A—C8 | 52.5 (5) | | |
Hydrogen-bond geometry (Å, º) top D—H···A | D—H | H···A | D···A | D—H···A |
N3—HN3A···Cl1 | 0.93 (2) | 2.40 (2) | 3.2245 (17) | 148.7 (18) |
N3—HN3B···Cl1i | 0.841 (19) | 2.41 (2) | 3.1948 (17) | 156.0 (17) |
Symmetry code: (i) −x+1/2, y−1/2, −z+1/2. |
[2-({[2-(Piperazin-1-yl-
κ2N1,
N4)ethyl]imino-
κN}methyl)benzenethiolato-
κS]nickel(II) hexafluorophosphate dichloromethane monosolvate (2)
top Crystal data top [Ni(C13H18N3S)]PF6·CH2Cl2 | Z = 2 |
Mr = 536.97 | F(000) = 544 |
Triclinic, P1 | Dx = 1.788 Mg m−3 |
a = 8.725 (3) Å | Mo Kα radiation, λ = 0.71075 Å |
b = 10.507 (4) Å | Cell parameters from 3095 reflections |
c = 11.316 (4) Å | θ = 3.3–27.5° |
α = 98.065 (4)° | µ = 1.49 mm−1 |
β = 101.274 (6)° | T = 153 K |
γ = 96.150 (5)° | Prism, orange |
V = 997.6 (6) Å3 | 0.17 × 0.11 × 0.08 mm |
Data collection top Rigaku AFC11 with Saturn 724+ CCD diffractometer | 4392 independent reflections |
Radiation source: Rotating Anode | 3240 reflections with I > 2σ(I) |
Detector resolution: 28.5714 pixels mm-1 | Rint = 0.041 |
ω scans | θmax = 27.5°, θmin = 3.3° |
Absorption correction: multi-scan (REQAB; Rigaku, 1998) | h = −10→11 |
Tmin = 0.828, Tmax = 1.000 | k = −13→13 |
8155 measured reflections | l = −14→11 |
Refinement top Refinement on F2 | Primary atom site location: structure-invariant direct methods |
Least-squares matrix: full | Hydrogen site location: difference Fourier map |
R[F2 > 2σ(F2)] = 0.037 | All H-atom parameters refined |
wR(F2) = 0.087 | w = 1/[σ2(Fo2) + (0.0389P)2] where P = (Fo2 + 2Fc2)/3 |
S = 0.93 | (Δ/σ)max = 0.001 |
4392 reflections | Δρmax = 0.79 e Å−3 |
333 parameters | Δρmin = −0.52 e Å−3 |
0 restraints | |
Special details top Geometry. All esds (except the esd in the dihedral angle between two l.s. planes) are estimated using the full covariance matrix. The cell esds are taken into account individually in the estimation of esds in distances, angles and torsion angles; correlations between esds in cell parameters are only used when they are defined by crystal symmetry. An approximate (isotropic) treatment of cell esds is used for estimating esds involving l.s. planes. |
Fractional atomic coordinates and isotropic or equivalent isotropic displacement parameters (Å2) top | x | y | z | Uiso*/Ueq | |
Ni1 | 0.57594 (4) | 0.27835 (3) | 0.57343 (3) | 0.01844 (11) | |
Cl1 | 0.25537 (9) | 0.20611 (8) | 0.80312 (7) | 0.0387 (2) | |
Cl2 | 0.11870 (9) | 0.29079 (8) | 1.01271 (7) | 0.03346 (19) | |
S1 | 0.32922 (8) | 0.21518 (7) | 0.51052 (6) | 0.02276 (16) | |
P1 | 0.72668 (8) | 0.35508 (8) | 0.17761 (7) | 0.02436 (18) | |
F1 | 0.5860 (3) | 0.2992 (3) | 0.2304 (3) | 0.0855 (9) | |
F2 | 0.6694 (3) | 0.2481 (2) | 0.0598 (2) | 0.0743 (8) | |
F3 | 0.8640 (3) | 0.4110 (3) | 0.1214 (3) | 0.0879 (9) | |
F4 | 0.7800 (3) | 0.4640 (3) | 0.2946 (2) | 0.0931 (10) | |
F5 | 0.8362 (2) | 0.2621 (2) | 0.24263 (19) | 0.0551 (6) | |
F6 | 0.6156 (2) | 0.44975 (18) | 0.11403 (16) | 0.0351 (4) | |
N1 | 0.6501 (2) | 0.1584 (2) | 0.4715 (2) | 0.0199 (5) | |
N2 | 0.7907 (2) | 0.3602 (2) | 0.6333 (2) | 0.0213 (5) | |
N3 | 0.5633 (3) | 0.4116 (2) | 0.7042 (2) | 0.0241 (5) | |
C1 | 0.2997 (3) | 0.1243 (3) | 0.3646 (3) | 0.0203 (6) | |
C2 | 0.1451 (3) | 0.1044 (3) | 0.2930 (3) | 0.0279 (7) | |
C3 | 0.1064 (4) | 0.0296 (3) | 0.1791 (3) | 0.0323 (7) | |
C4 | 0.2205 (4) | −0.0298 (3) | 0.1305 (3) | 0.0336 (8) | |
C5 | 0.3726 (4) | −0.0106 (3) | 0.1968 (3) | 0.0286 (7) | |
C6 | 0.4164 (3) | 0.0657 (3) | 0.3139 (3) | 0.0224 (6) | |
C7 | 0.5804 (3) | 0.0807 (3) | 0.3730 (3) | 0.0222 (6) | |
C8 | 0.8213 (3) | 0.1579 (3) | 0.5183 (3) | 0.0255 (6) | |
C9 | 0.8952 (3) | 0.2982 (3) | 0.5619 (3) | 0.0261 (7) | |
C10 | 0.8201 (4) | 0.3475 (3) | 0.7658 (3) | 0.0297 (7) | |
C11 | 0.6707 (4) | 0.3764 (3) | 0.8096 (3) | 0.0302 (7) | |
C12 | 0.7813 (4) | 0.4991 (3) | 0.6233 (3) | 0.0259 (6) | |
C13 | 0.6363 (4) | 0.5334 (3) | 0.6723 (3) | 0.0272 (7) | |
C14 | 0.2908 (4) | 0.2570 (4) | 0.9634 (3) | 0.0385 (8) | |
H2 | 0.070 (3) | 0.152 (3) | 0.325 (3) | 0.034 (9)* | |
H3 | 0.002 (3) | 0.016 (3) | 0.134 (3) | 0.027 (8)* | |
H3N | 0.479 (3) | 0.421 (3) | 0.719 (3) | 0.019 (8)* | |
H4 | 0.194 (3) | −0.085 (3) | 0.047 (3) | 0.037 (9)* | |
H5 | 0.451 (3) | −0.054 (3) | 0.168 (3) | 0.032 (9)* | |
H7 | 0.639 (3) | 0.028 (3) | 0.332 (3) | 0.028 (8)* | |
H8A | 0.829 (3) | 0.111 (3) | 0.588 (3) | 0.028 (8)* | |
H8B | 0.869 (3) | 0.118 (3) | 0.452 (3) | 0.027 (8)* | |
H9A | 1.006 (3) | 0.303 (3) | 0.611 (3) | 0.025 (8)* | |
H9B | 0.902 (3) | 0.344 (3) | 0.496 (3) | 0.024 (8)* | |
H10A | 0.841 (3) | 0.260 (3) | 0.770 (2) | 0.015 (7)* | |
H10B | 0.913 (3) | 0.404 (3) | 0.812 (3) | 0.029 (8)* | |
H11A | 0.621 (3) | 0.303 (3) | 0.834 (2) | 0.010 (6)* | |
H11B | 0.687 (3) | 0.448 (3) | 0.878 (3) | 0.033 (9)* | |
H12A | 0.877 (3) | 0.559 (3) | 0.667 (3) | 0.025 (8)* | |
H12B | 0.775 (3) | 0.514 (3) | 0.539 (3) | 0.025 (8)* | |
H13A | 0.667 (3) | 0.602 (3) | 0.743 (3) | 0.020 (7)* | |
H13B | 0.565 (3) | 0.566 (3) | 0.616 (3) | 0.019 (7)* | |
H14A | 0.324 (4) | 0.184 (4) | 1.000 (3) | 0.052 (11)* | |
H14B | 0.365 (4) | 0.337 (4) | 0.982 (4) | 0.064 (13)* | |
Atomic displacement parameters (Å2) top | U11 | U22 | U33 | U12 | U13 | U23 |
Ni1 | 0.01714 (19) | 0.01885 (19) | 0.01872 (19) | 0.00118 (13) | 0.00293 (14) | 0.00337 (14) |
Cl1 | 0.0409 (5) | 0.0399 (5) | 0.0342 (4) | 0.0054 (4) | 0.0098 (4) | −0.0002 (4) |
Cl2 | 0.0305 (4) | 0.0396 (5) | 0.0276 (4) | −0.0010 (3) | 0.0044 (3) | 0.0036 (3) |
S1 | 0.0184 (3) | 0.0259 (4) | 0.0240 (4) | 0.0026 (3) | 0.0050 (3) | 0.0039 (3) |
P1 | 0.0214 (4) | 0.0288 (4) | 0.0229 (4) | 0.0044 (3) | 0.0037 (3) | 0.0049 (3) |
F1 | 0.0698 (15) | 0.098 (2) | 0.136 (2) | 0.0414 (15) | 0.0698 (17) | 0.0879 (19) |
F2 | 0.1012 (18) | 0.0459 (15) | 0.0566 (15) | 0.0286 (13) | −0.0211 (13) | −0.0173 (12) |
F3 | 0.0450 (13) | 0.111 (2) | 0.137 (3) | 0.0190 (14) | 0.0501 (16) | 0.069 (2) |
F4 | 0.134 (2) | 0.0682 (18) | 0.0475 (15) | 0.0435 (17) | −0.0427 (15) | −0.0239 (14) |
F5 | 0.0545 (13) | 0.0671 (16) | 0.0495 (13) | 0.0363 (11) | 0.0028 (10) | 0.0201 (12) |
F6 | 0.0394 (10) | 0.0343 (11) | 0.0340 (10) | 0.0125 (8) | 0.0044 (8) | 0.0123 (8) |
N1 | 0.0178 (11) | 0.0194 (12) | 0.0234 (12) | 0.0029 (9) | 0.0028 (10) | 0.0086 (10) |
N2 | 0.0187 (12) | 0.0214 (12) | 0.0224 (12) | −0.0008 (9) | 0.0001 (10) | 0.0073 (10) |
N3 | 0.0237 (14) | 0.0268 (14) | 0.0212 (12) | 0.0011 (11) | 0.0052 (11) | 0.0034 (10) |
C1 | 0.0198 (14) | 0.0174 (14) | 0.0248 (15) | 0.0019 (11) | 0.0041 (12) | 0.0087 (11) |
C2 | 0.0237 (16) | 0.0267 (16) | 0.0320 (17) | 0.0016 (13) | 0.0006 (14) | 0.0098 (13) |
C3 | 0.0281 (17) | 0.0295 (18) | 0.0336 (18) | −0.0037 (13) | −0.0073 (15) | 0.0117 (14) |
C4 | 0.045 (2) | 0.0236 (16) | 0.0254 (16) | −0.0033 (14) | −0.0047 (15) | 0.0028 (13) |
C5 | 0.0341 (18) | 0.0218 (15) | 0.0278 (16) | 0.0025 (13) | 0.0032 (14) | 0.0026 (13) |
C6 | 0.0242 (15) | 0.0171 (14) | 0.0252 (15) | 0.0000 (11) | 0.0034 (12) | 0.0063 (12) |
C7 | 0.0258 (16) | 0.0170 (14) | 0.0275 (15) | 0.0060 (12) | 0.0105 (13) | 0.0066 (12) |
C8 | 0.0183 (15) | 0.0273 (16) | 0.0319 (17) | 0.0066 (12) | 0.0037 (13) | 0.0079 (14) |
C9 | 0.0184 (15) | 0.0281 (17) | 0.0325 (17) | 0.0015 (12) | 0.0047 (13) | 0.0094 (14) |
C10 | 0.0295 (17) | 0.0324 (18) | 0.0230 (16) | −0.0022 (14) | −0.0059 (13) | 0.0115 (14) |
C11 | 0.0401 (19) | 0.0314 (18) | 0.0177 (15) | 0.0002 (14) | 0.0036 (14) | 0.0069 (13) |
C12 | 0.0256 (16) | 0.0237 (16) | 0.0271 (16) | −0.0027 (12) | 0.0036 (13) | 0.0078 (13) |
C13 | 0.0350 (18) | 0.0225 (16) | 0.0221 (15) | 0.0021 (13) | 0.0018 (14) | 0.0042 (13) |
C14 | 0.0293 (18) | 0.051 (2) | 0.0314 (19) | 0.0023 (17) | −0.0002 (15) | 0.0072 (17) |
Geometric parameters (Å, º) top Ni1—N1 | 1.843 (2) | C3—C4 | 1.391 (5) |
Ni1—N3 | 1.917 (3) | C3—H3 | 0.94 (3) |
Ni1—N2 | 1.924 (2) | C4—C5 | 1.369 (4) |
Ni1—S1 | 2.1316 (10) | C4—H4 | 1.01 (3) |
Cl1—C14 | 1.773 (4) | C5—C6 | 1.408 (4) |
Cl2—C14 | 1.755 (4) | C5—H5 | 0.95 (3) |
S1—C1 | 1.745 (3) | C6—C7 | 1.438 (4) |
P1—F3 | 1.561 (2) | C7—H7 | 0.93 (3) |
P1—F1 | 1.562 (2) | C8—C9 | 1.517 (4) |
P1—F2 | 1.578 (2) | C8—H8A | 0.98 (3) |
P1—F4 | 1.581 (2) | C8—H8B | 0.99 (3) |
P1—F5 | 1.592 (2) | C9—H9A | 1.01 (3) |
P1—F6 | 1.6064 (19) | C9—H9B | 0.95 (3) |
N1—C7 | 1.285 (3) | C10—C11 | 1.528 (5) |
N1—C8 | 1.485 (3) | C10—H10A | 0.96 (3) |
N2—C9 | 1.476 (4) | C10—H10B | 0.96 (3) |
N2—C12 | 1.489 (4) | C11—H11A | 0.94 (3) |
N2—C10 | 1.499 (4) | C11—H11B | 0.98 (3) |
N3—C11 | 1.482 (4) | C12—C13 | 1.535 (4) |
N3—C13 | 1.490 (4) | C12—H12A | 0.98 (3) |
N3—H3N | 0.80 (3) | C12—H12B | 0.98 (3) |
C1—C2 | 1.409 (4) | C13—H13A | 0.97 (3) |
C1—C6 | 1.417 (4) | C13—H13B | 0.93 (3) |
C2—C3 | 1.371 (4) | C14—H14A | 0.96 (4) |
C2—H2 | 0.96 (3) | C14—H14B | 0.97 (4) |
| | | |
N1—Ni1—N3 | 162.95 (10) | C4—C5—H5 | 120.6 (18) |
N1—Ni1—N2 | 87.80 (10) | C6—C5—H5 | 117.3 (18) |
N3—Ni1—N2 | 76.05 (10) | C5—C6—C1 | 119.0 (3) |
N1—Ni1—S1 | 98.90 (7) | C5—C6—C7 | 116.6 (3) |
N3—Ni1—S1 | 97.67 (8) | C1—C6—C7 | 124.4 (3) |
N2—Ni1—S1 | 171.77 (7) | N1—C7—C6 | 127.1 (3) |
C1—S1—Ni1 | 107.93 (9) | N1—C7—H7 | 119.2 (18) |
F3—P1—F1 | 178.42 (16) | C6—C7—H7 | 113.7 (18) |
F3—P1—F2 | 89.42 (17) | N1—C8—C9 | 107.1 (2) |
F1—P1—F2 | 89.56 (17) | N1—C8—H8A | 105.7 (16) |
F3—P1—F4 | 91.08 (18) | C9—C8—H8A | 110.2 (18) |
F1—P1—F4 | 89.91 (18) | N1—C8—H8B | 109.8 (16) |
F2—P1—F4 | 178.47 (14) | C9—C8—H8B | 110.4 (17) |
F3—P1—F5 | 90.76 (14) | H8A—C8—H8B | 113 (2) |
F1—P1—F5 | 90.47 (13) | N2—C9—C8 | 106.7 (2) |
F2—P1—F5 | 91.00 (13) | N2—C9—H9A | 112.5 (16) |
F4—P1—F5 | 90.44 (13) | C8—C9—H9A | 110.4 (17) |
F3—P1—F6 | 89.75 (13) | N2—C9—H9B | 107.6 (17) |
F1—P1—F6 | 89.03 (12) | C8—C9—H9B | 112.4 (18) |
F2—P1—F6 | 89.73 (12) | H9A—C9—H9B | 107 (2) |
F4—P1—F6 | 88.82 (12) | N2—C10—C11 | 106.3 (2) |
F5—P1—F6 | 179.11 (11) | N2—C10—H10A | 106.5 (16) |
C7—N1—C8 | 118.7 (2) | C11—C10—H10A | 112.9 (16) |
C7—N1—Ni1 | 131.57 (19) | N2—C10—H10B | 111.7 (18) |
C8—N1—Ni1 | 109.78 (18) | C11—C10—H10B | 112.7 (17) |
C9—N2—C12 | 114.7 (2) | H10A—C10—H10B | 107 (2) |
C9—N2—C10 | 115.9 (2) | N3—C11—C10 | 106.3 (2) |
C12—N2—C10 | 108.4 (2) | N3—C11—H11A | 109.2 (16) |
C9—N2—Ni1 | 110.28 (17) | C10—C11—H11A | 111.3 (16) |
C12—N2—Ni1 | 103.48 (17) | N3—C11—H11B | 107.8 (19) |
C10—N2—Ni1 | 102.70 (17) | C10—C11—H11B | 114.8 (17) |
C11—N3—C13 | 109.2 (2) | H11A—C11—H11B | 107 (2) |
C11—N3—Ni1 | 102.14 (19) | N2—C12—C13 | 106.3 (2) |
C13—N3—Ni1 | 104.61 (18) | N2—C12—H12A | 114.1 (17) |
C11—N3—H3N | 111 (2) | C13—C12—H12A | 111.2 (16) |
C13—N3—H3N | 110 (2) | N2—C12—H12B | 111.3 (18) |
Ni1—N3—H3N | 119 (2) | C13—C12—H12B | 112.4 (17) |
C2—C1—C6 | 117.5 (3) | H12A—C12—H12B | 102 (2) |
C2—C1—S1 | 116.8 (2) | N3—C13—C12 | 106.1 (3) |
C6—C1—S1 | 125.6 (2) | N3—C13—H13A | 111.2 (17) |
C3—C2—C1 | 121.9 (3) | C12—C13—H13A | 110.8 (16) |
C3—C2—H2 | 121.7 (18) | N3—C13—H13B | 111.6 (17) |
C1—C2—H2 | 116.2 (18) | C12—C13—H13B | 112.1 (17) |
C2—C3—C4 | 120.4 (3) | H13A—C13—H13B | 105 (2) |
C2—C3—H3 | 120.1 (17) | Cl2—C14—Cl1 | 112.25 (19) |
C4—C3—H3 | 119.5 (17) | Cl2—C14—H14A | 106 (2) |
C5—C4—C3 | 119.1 (3) | Cl1—C14—H14A | 106 (2) |
C5—C4—H4 | 119.4 (17) | Cl2—C14—H14B | 108 (2) |
C3—C4—H4 | 121.5 (17) | Cl1—C14—H14B | 107 (2) |
C4—C5—C6 | 121.9 (3) | H14A—C14—H14B | 117 (3) |
| | | |
N3—Ni1—N1—C7 | −178.7 (3) | C5—C6—C7—N1 | 170.2 (3) |
N2—Ni1—N1—C7 | −160.0 (3) | C1—C6—C7—N1 | −8.5 (5) |
S1—Ni1—N1—C7 | 15.1 (3) | C7—N1—C8—C9 | 138.9 (3) |
N3—Ni1—N1—C8 | 1.5 (5) | Ni1—N1—C8—C9 | −41.2 (3) |
N2—Ni1—N1—C8 | 20.09 (19) | C12—N2—C9—C8 | −146.0 (2) |
S1—Ni1—N1—C8 | −164.72 (18) | C10—N2—C9—C8 | 86.4 (3) |
Ni1—S1—C1—C2 | −162.6 (2) | Ni1—N2—C9—C8 | −29.6 (3) |
Ni1—S1—C1—C6 | 19.5 (3) | N1—C8—C9—N2 | 45.1 (3) |
C6—C1—C2—C3 | 1.1 (4) | C9—N2—C10—C11 | −161.6 (2) |
S1—C1—C2—C3 | −177.0 (2) | C12—N2—C10—C11 | 67.7 (3) |
C1—C2—C3—C4 | 0.1 (5) | Ni1—N2—C10—C11 | −41.3 (3) |
C2—C3—C4—C5 | −1.3 (5) | C13—N3—C11—C10 | −64.2 (3) |
C3—C4—C5—C6 | 1.1 (5) | Ni1—N3—C11—C10 | 46.2 (3) |
C4—C5—C6—C1 | 0.1 (5) | N2—C10—C11—N3 | −2.9 (3) |
C4—C5—C6—C7 | −178.7 (3) | C9—N2—C12—C13 | 164.6 (2) |
C2—C1—C6—C5 | −1.2 (4) | C10—N2—C12—C13 | −64.2 (3) |
S1—C1—C6—C5 | 176.7 (2) | Ni1—N2—C12—C13 | 44.4 (3) |
C2—C1—C6—C7 | 177.5 (3) | C11—N3—C13—C12 | 67.6 (3) |
S1—C1—C6—C7 | −4.7 (4) | Ni1—N3—C13—C12 | −41.1 (3) |
C8—N1—C7—C6 | 179.4 (3) | N2—C12—C13—N3 | −2.2 (3) |
Ni1—N1—C7—C6 | −0.4 (5) | | |
Hydrogen-bond geometry (Å, º) topCg6 is the centroid of the C1–C6 ring. |
D—H···A | D—H | H···A | D···A | D—H···A |
N3—H3N···F6i | 0.80 (3) | 2.50 (3) | 3.114 (3) | 135 (3) |
C10—H10A···C4ii | 0.96 (3) | 2.85 (3) | 0.0000 (5) | 147 (2) |
C8—H8A···Cg6ii | 0.98 (3) | 2.84 (3) | 3.778 (4) | 160 (2) |
Symmetry codes: (i) −x+1, −y+1, −z+1; (ii) −x+1, −y, −z+1. |
References
Altomare, A., Burla, M. C., Camalli, M., Cascarano, G. L., Giacovazzo, C., Guagliardi, A., Moliterni, A. G. G., Polidori, G. & Spagna, R. (1999). J. Appl. Cryst. 32, 115–119. Web of Science CrossRef CAS IUCr Journals Google Scholar
Bouwman, E., Henderson, R. K., Reedijk, J., Veldman, N. & Spek, A. L. (1999). Inorg. Chim. Acta, 287, 105–108. CSD CrossRef CAS Google Scholar
Farrugia, L. J. (2012). J. Appl. Cryst. 45, 849–854. Web of Science CrossRef CAS IUCr Journals Google Scholar
Feldscher, B., Theil, H., Stammler, A., Bögge, H. & Glaser, T. (2014). Dalton Trans. 43, 4102–4114. CSD CrossRef CAS PubMed Google Scholar
Goswami, N. & Eichhorn, D. M. (1999). Inorg. Chem. 38, 4329–4333. Web of Science CSD CrossRef CAS Google Scholar
Han, Z., McNamara, W. R., Eum, M.-S., Holland, P. L. & Eisenberg, R. (2012). Angew. Chem. Int. Ed. 51, 1667–1670. Web of Science CrossRef CAS Google Scholar
Helm, M. L., Stewart, M. P., Bullock, M., DuBois, M. R. & DuBois, D. L. (2011). Science, 333, 863–866. CSD CrossRef CAS PubMed Google Scholar
Inoue, S., Yan, Y.-N., Yamanishi, K., Kataoka, Y. & Kawamoto, T. (2020). Chem. Commun. 56, 2829–2832. CSD CrossRef CAS Google Scholar
Lubitz, W., Ogata, H., Rüdiger, O. & Reijerse, E. (2014). Chem. Rev. 114, 4081–4148. Web of Science CrossRef CAS PubMed Google Scholar
Luo, G.-G., Wang, Y.-H., Wang, J.-H., Wu, J.-H. & Wu, R. (2017). Chem. Commun. 53, 7007–7010. CrossRef CAS Google Scholar
Macrae, C. F., Sovago, I., Cottrell, S. J., Galek, P. T. A., McCabe, P., Pidcock, E., Platings, M., Shields, G. P., Stevens, J. S., Towler, M. & Wood, P. A. (2020). J. Appl. Cryst. 53, 226–235. Web of Science CrossRef CAS IUCr Journals Google Scholar
Martin, D. J., McCarthy, B. D., Donley, C. L. & Dempsey, J. L. (2015). Chem. Commun. 51, 5290–5293. CrossRef CAS Google Scholar
Mukhopadhyay, S., Mandal, D., Ghosh, D., Goldberg, I. & Chaudhury, M. (2003). Inorg. Chem. 42, 8439–8445. Web of Science CSD CrossRef PubMed CAS Google Scholar
Rigaku (1998). REQAB, Rigaku Corporation, Tokyo, Japan. Google Scholar
Rigaku (2008). CrystalClear, Rigaku Corporation, Tokyo, Japan. Google Scholar
Sheldrick, G. M. (2015). Acta Cryst. C71, 3–8. Web of Science CrossRef IUCr Journals Google Scholar
Stewart, M. P., Ho, M.-H., Wiese, S., Lindstrom, M. L., Thogerson, C. E., Raugei, S., Bullock, R. M. & Helm, M. L. (2013). J. Am. Chem. Soc. 135, 6033–6046. Web of Science CSD CrossRef CAS PubMed Google Scholar
Yamamura, T., Tadokoro, M., Tanaka, K. & Kuroda, R. (1993). Bull. Chem. Soc. Jpn, 66, 1984–1990. CSD CrossRef CAS Google Scholar
This is an open-access article distributed under the terms of the Creative Commons Attribution (CC-BY) Licence, which permits unrestricted use, distribution, and reproduction in any medium, provided the original authors and source are cited.
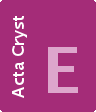 | CRYSTALLOGRAPHIC COMMUNICATIONS |
ISSN: 2056-9890
Open

access