1. Accelerators
The UVSOR accelerator complex consists of a 15 MeV injector linac, a 600 MeV booster synchrotron, and a 750 MeV storage ring. The storage-ring room and the synchrotron room are constructed separately on the second underground floor. Fig. 1
shows a picture of the UVSOR storage ring. The magnet lattice of the storage ring is the so-called double-bend achromat. The main parameters of the accelerators are listed in Table 1
. A harmonic cavity was commissioned in Spring 1993 to suppress longitudinal coupled-bunch instability by Landau damping. The double RF system is routinely operated for user beam time, and the lifetime of the beam has been improved to about 5 h at 200 mA.
Energy | 15 MeV | Energy spread | 1.6% | Frequency | S-band 2.856 GHz | Acceleration | 2π/3 travelling wave | Length | 2.5 m (from gun to exit) | Klystron power | 1.8 MW | | Lattice type | FODO | Energy | 600 MeV | Beam current | 32 mA (8-bunch filled) | Circumference | 26.6 m | Supercell | 6 | Bending radius | 1.8 m | Betatron number | 2.25 (horizontal) | | 1.25 (vertical) | Momentum compaction, α | 0.138 | Harmonics | 8 | RF frequency | 90.115 MHz | Repetition rate | 2.6 Hz | | Lattice type | Chasman–Green | Energy | 750 MeV | Critical energy | 425 eV | Circumference | 53.2 m | Super cell | 4 | Bending radius | 2.2 m | Betatron tune | 3.16 (horizontal) | | 2.64 (vertical) | Momentum compaction, α | 0.032 | Harmonics | 16 | RF frequency | 90.115 MHz | RF voltage | 50 kV | Emittance | 115π nm rad (horizontal) | | 11.5π nm rad (vertical) | Beam size | 0.39 mm (horizontal) | | 0.27 mm (vertical) | Bunch length | 170 ps (at zero current) | Initial beam current | 200 mA (multibunch) | | 50 mA (single bunch) | Lifetime (multibunch) | 9 h at 100 mA | | |
The storage ring was divided into four sections by gate valves in Spring 1995 in order to make a scheduled shutdown easier. The baking system was also replaced by a new one which included controllable indirect heating. The RF-power amplifier systems of the booster synchrotron and the storage ring were replaced by solid-state systems in 1996 in order to obtain more stable operation and easier maintenance.
The storage ring is normally operated under multi-bunch mode with partial filling. Single-bunch operation is also conducted for about four weeks in a year to provide pulsed synchrotron radiation for time-resolved experiments. A resonance transverse kicker was installed in Spring 1997 to maintain the bunch purity and to study phase dynamics. Typical beam currents under multibunch and single-bunch modes are 200 mA and 50 mA, respectively.
2. Insertion devices
The UVSOR storage ring consists of eight bending magnets and three insertion devices. The bending magnet of radius 2.2 m provides the synchrotron radiation, the critical energy of which is 425 eV. Table 2
shows the main parameters of the insertion devices. The superconducting wiggler is a wavelength-shifter type (Nakamura et al., 1996
). The 4 T magnetic field can provide soft X-rays up to 6 keV. It is used for about half of the user beam time. A planar undulator consists of 24 pairs of permanent magnets, the period of which is 84 mm. The fundamentals from the undulator provide quasi-monochromatic intense radiation in the range 8–52 eV. Since the K value changes from 0.6 to 3.6, higher harmonics are mixed into the spectral distribution in the case of high K values. A helical undulator was installed into one of the four straight sections of the storage ring in 1996 to provide circularly polarized light in the energy range 5–250 eV (Kimura et al., 1996
). An optional version of the helical undulator is a helical optical klystron, which is powerful for a free-electron-laser (FEL) experiment (Hama, 1996
). The helical undulator consists of two sets of permanent magnets producing a vertical magnetic field and four sets of permanent magnets making a horizontal magnetic field. The four sets of magnets can move in the transverse direction to switch the helicity of the circular polarization. The undulator consists of permanent magnets having 18 periods, a period length of 110 mm, and remanent field of 1.3 T, and a dispersive part having one period and a period length of 302.5 mm. The K value changes from 0.07 to 4.6 and from 0.15 to 8.5 for helical and planar modes, respectively. The helical undulator can provide perfectly circular polarization in the range of 2–45 eV, and elliptical polarization up to 200 eV. The intensity distribution of the helical undulator is shown in Fig. 2
.
Type | Wavelength-shifter type | Maximum magnetic field | 4 T | Magnetic gap | 45 mm | Critical energy | 1.5 keV | | Number of periods | 24 | Period length, λu | 84 mm | Total length | 2016 mm | Remanent field, Br | 0.9 T | Vertical gap of magnets | 30–90 mm | Deflection parameter, K | 0.6–3.6 | Photon energy of fundamentals | 8–52 eV | | Number of periods | 18 | Period length, λu | 110 mm | Length of dispersive part | 302.5 mm | Total length | 2351.2 mm | Remanent field, Br | 1.3 T | Vertical gap of magnets | 30–150 mm | Deflection parameter, Kx,y | 0.07–4.6 (helical mode) | Deflection parameter, K | 0.15–8.5 (planar mode) | Photon energy of fundamentals | 2–45 eV (circular polarization) | | |
| Figure 2 Intensity distribution of the helical undulator. |
3. Beamlines
Initially, 15 beamlines were constructed giving priority to studies of four fields: `spectroscopy', `photoelectron spectroscopy', `photochemical reactions' and `elementary processes of chemical reactions'. Several years later, four beamlines were added to expand the research to the fields `solid and surface photochemical reaction' and `photochemical material processing'. In recent years, `combination of synchrotron radiation with laser beams' was proposed to be added to the important research fields.
The UVSOR group have discussed the improvements and upgrades of the beamlines with users. Workshops on VUV beamlines for solid-state research, on beamlines for soft X-rays, chemical reactions and gas phases, and on beamlines for the IR and far-IR regions were held in 1994, 1995 and 1996, respectively. About one-third of beamlines have been upgraded over a couple of years. A Seya–Namioka monochromator at beamline 7B is being replaced by a normal-incidence monochromator (NIM) to improve the resolving power and spectral range for solid-state spectroscopy. Another Seya–Namioka monochromator at beamline 2B2 is also being replaced by a Dragon-type monochromator for gaseous experiments in VUV and EUV ranges. A multilayer monochromator is being installed into beamline 4A for photochemical reaction experiments. A glancing-incidence monochromator at BL8B1 has been replaced by a 15 m SGM monochromator (Hiraya et al., 1995
). This new monochromator, having a resolving power of 4000 at 400 eV, is used for solid-state and gaseous experiments in the EUV region. A Bruker FT-IR interferometer has been installed into beamline 6A1 beside the old Martin–Puplett-type FT-FIR; the improved system can cover the wide wavelength range from 1 µm to 3 mm. A new monochromator (SGM-TRAIN) has been constructed at beamline 5A for the use of circularly polarized light from the helical undulator (Kamada et al., 1995
). As shown in Fig. 3
, the SGM-TRAIN consists of 7.5 m and 25 m CL-SGM's and a 2.5 m NIM, and is expected to provide a resolving power of 10000 at 100 eV. As shown in Table 3
, the UVSOR has two soft X-ray stations equipped with a double-crystal monochromator, eight EUV stations with a glancing-incidence or a plane-grating monochromator, four VUV stations with a Seya–Namioka-type or a normal-incidence-type monochromator, two (far) IR stations equipped with FT interferometers, a multilayer monochromator, and three white-light stations without a monochromator.
Beamline | Monochromator, spectrometer | Wavelength region | Acceptance angle (mrad) Horizontal/Vertical | Experiment | BL1A | Double-crystal | 2.1–0.3 nm | 4/1 | Solid (photoemission) | BL1B | 1 m Seya–Namioka | 650–30 nm | 60/6 | Solid (absorption) | BL2A | 1 m Seya–Namioka | 400–30 nm | 40/6 | Gas (absorption) | BL2B1 | 2 m Grasshopper | 60–1.5 nm | 10/1.7 | Solid and surface (photoemisson) | BL2B2 | Dragon | 60–6 nm | 15/6 | Gas (photoionization, photodissociation) | BL3A1 | None (filter, mirror) | (U) | 0.3/0.3 | Solid and irradiation (photodissociation) | BL3A2 | 2.2 m constant-deviation grazing incidence | 100–10 nm (U) | 10/4 | Gas and solid (photoionization, | | | | 0.3/0.3 | photodissociation) | BL3B | 3 m normal incidence | 400–30 nm | 20/6 | Gas (photoemission) | BL4A | Multilayer | 83–18 nm | 16.5/12.8 | Irradiation | BL4B | None | | 8.3/6 | Irradiation | BL5A | None | (OK) | | FEL | | SGM-TRAIN | 250–5 nm | 10/3 | Solid (photoemission) | BL5B | Plane grating | 200–2 nm | 10/2.2 | Calibration, gas (photodissociation) and solid (absorption) | BL6A1 | Martin-Puplett FT-IR | 3000–30 mm | 80/60 | Solid (absorption) | | Michelson FT-IR | 100–1 mm | 80/60 | | BL6A2 | Plane grating | 650–8 nm | 10/6 | Solid and surface (photoemission) | BL6B | FT-IR | 200–1.7 mm | 70/25 | Solid (absorption) | BL7A | Double crystal | 1.5–0.8 nm | 2/0.3 | Solid (absorption) | | | 1.5–0.2 nm (W) | 1/0.15 | | BL7B | 3 m normal incidence | 1000–50 nm | 45/10.5 | Solid (absorption) | BL8A | None (filter) | | 25/8 | Irradiation and user instrumentation | BL8B1 | 15 m constant-deviation grazing incidence | 40–2 nm | 10/1.5 | Gas and solid (absorption) | BL8B2 | Plane grating | 650–8 nm | 10/6 | Solid (photoemission) | | |
Photoelectron spectroscopy combined with synchrotron radiation is one of the most powerful techniques for investigating electronic structures. Several kinds of photoelectron spectrometers are working or are ready to work in UVSOR: a high-performance electron analyser at soft X-ray beamline 1A, a two-dimensional spectrometer at VUV beamline 3B, an angle-resolved spectrometer at EUV beamlines 6A2 and 8B2, a photoelectron microscopy system at wiggler beamline 7A (sometimes this system can be used at another beamline, 5B), and a spin- and angle-resolved photoelectron spectrometer at helical undulator beamline 5A.
4. Research activities
Several trials with much effort have been carried out in recent years to achieve new scientific and experimental findings. Scientific investigations of FELs have been conducted, and a UV FEL of 239 nm was obtained in October 1996. New experiments on solid surfaces with an electron–ion coincidence (EICO) method have started at beamline 2B1. Laser and synchrotron radiation combination experiments for pump–probe and double resonance have also been conducted in the solid and gas phases at beamlines 1B, 3A1 and 3A2. A high-sensitivity detection system consisting of a conventional analysing monochromator and a CCD detector has been available at beamline 1B to observe luminescence in organic and inorganic materials. A multi-gate system combined with a time-correlated single-photon counting method makes it possible to observe three time-resolved spectra simultaneously under a single-bunch operation. Moreover, synchrotron radiation offers the advantages of a brilliant broad-band light source in the far-IR region. A highest pressure of 20 GPa in the far-IR region was achieved at beamline 6A1 by using a diamond-anvil-cell technique. Photochemical reaction experiments on Si, diamond and halides, soft X-ray microscopy on biospecimens, and incoherent photon-echo experiments have been successfully carried out at beamlines 4B, 3A1 and 8A. Details are given in the UVSOR Activity Report, which is published annually.
5. Summary
The UVSOR has been open to Japanese universities and national institutes for nearly 14 years. The scientific programs cover a variety of fields, including molecular science, physics, chemistry, biology, engineering, and so on. In the fiscal year 1996, three subject-research programs, 26 collaboration-research programs and 149 facility-use programs have been conducted. The number of users was about 600 including scientists from foreign countries. The machine and beamlines have been improved in recent years. Moreover, the UVSOR has started to think about the future. Discussions of machine design for the new UVSOR II began in 1995. Users also organized their group in 1995 in order to discuss the present and future plans as well as to present their requirements and proposals.
Those interested in updated information about the UVSOR facility are directed to http://www.uvsor.ims.ac.jp/ or http://133.48.172.35/beamline/beamline.htm.
References
Hama, H. (1996). Nucl. Instrum. Methods, A375, 57–61. CrossRef Web of Science
Hiraya, A., Nakamura, E., Hasumoto, M., Kinoshita, T., Sakai, K., Ishiguro, E. & Watanabe, M. (1995). Rev. Sci. Instrum. 66, 2104–2106. CrossRef CAS Web of Science
Kamada, M., Sakai, K., Tanaka, S., Ohara, S., Kimura, S., Hiraya, A., Hasumoto, M., Nakagawa, K., Ichikawa, K., Soda, K., Flukui, K., Flujii, Y. & Ishiguro, E. (1995). Rev. Sci. Instrum. 66, 1537–1539. CrossRef CAS Web of Science
Kimura S., Kamada, M., Hama, H., Mare´chal, X.-M., Tanaka, T. & Kitamura, H. (1996). J. Electron Spectrosc. Relat. Phenom. 80, 437–440.
Nakamura, E., Hama, H., Yamazaki, J., Kinoshita, T., Matsudo, O., Yonehara, H., Kasuga, T., Isoyama, G. & Watanabe, M. (1996). J. Electron Spectrosc. Relat. Phenom. 80, 421–424. CrossRef CAS Web of Science
© International Union of Crystallography. Prior permission is not required to reproduce short quotations, tables and figures from this article, provided the original authors and source are cited. For more information, click here.
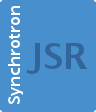 | JOURNAL OF SYNCHROTRON RADIATION |
ISSN: 1600-5775