1. Chemical context
Schiff base molecules are well-known ligands because of the ease of their formation and for their rich coordination chemistry with a wide range of metal ions. A prominent application of metal–Schiff base complexes is as catalysts in different chemical reactions (Patti et al., 2009
). The Schiff base molecules themselves are of considerable interest as they display a broad range of biological activities such as anti-bacterial, anti-fungal, anti-viral, anti-malarial, anti-inflammatory, etc. (Guo et al., 2007
; Przybylski et al., 2009
; Annapoorani & Krishnan, 2013
). A full range of metal complexes formed with these usually multidentate ligands often result in species with enhanced biological action (Bagihalli et al., 2008
; Tian et al., 2009
, 2011
; Chohan et al., 2001
). As part of our ongoing studies of Schiff base ligands and their metal complexes (Manawar, Gondaliya, Mamtora et al., 2019
), the crystal and molecular structures, Hirshfeld surface analysis and computational study of homoleptic CoII (I)
and CuII (II)
complexes derived from 2-{(1E)-[(E)-2-(2,6-dichlorobenzylidene)hydrazin-1-ylidene]methyl}phenol (Manawar, Gondaliya, Shah et al., 2019
) are described herein.
2. Structural commentary
The cobalt complex (I)
, Fig. 1
, lacks crystallographic symmetry and the metal ion is N,O-coordinated by two mono-anionic Schiff base ligands; selected geometric parameters are collated in Table 1
. The N2O2 donor set defines an approximate tetrahedron with the range of tetrahedral angles being over 30°. Thus, the narrowest angle of 94.06 (7)° is found for O1—Co—N1 while the widest of 125.33 (8)° is noted for O1—Co—O2. A geometric measure of a four-coordinate geometry is the value of τ4, which has values of τ4 = 1.0 for an ideal tetrahedron and τ4 = 0.0 for an ideal square-planar geometry (Yang et al., 2007
). In (I)
, τ4 = 0.82, indicating a geometry close to trigonal pyramidal. Each of the Schiff base ligands forms a six-membered Co,O,C3,N chelate ring. These adopt an envelope conformation with the Co atom lying 0.253 (3) Å out of the least-squares plane defined by the five remaining atoms of the O1-chelate ring (r.m.s. deviation = 0.0086 Å); the equivalent values for the O2-chelate ring are 0.376 (3) and 0.0222 Å, respectively. The dihedral angle formed between the planar regions of the chelate rings is 86.44 (8)°, consistent with a near to orthogonal relationship. For the O1-chelate ring, the dihedral angle between the five co-planar atoms and the fused-benzene and pendent dichlorobenzene rings are 0.92 (13) and 7.34 (14)°, respectively, and the dihedral angle between the benzene rings is 6.47 (15)°, indicating a small deviation from planarity. The equivalent dihedral angles for the O2-chelate ring are 1.99 (14), 7.25 (12) and 5.58 (12)°, respectively. These data are consistent with small twists about the N1—N2 [the C7—N1—N2—C8 torsion angle = 166.6 (2)°] and C16—C21 [C15—C16—C21—N3 = 6.4 (4)°] bonds. Finally, each Schiff base ligand features two imine bonds, with the bond length involving the coordinated N1 atom [1.304 (3) Å] being longer than the second N2-imine bond [1.251 (3) Å]; for the O2-ligand, C21—N3 = 1.303 (3) Å and C22—N4 = 1.247 (3) Å. The configurations about imine bonds are different with those involving the coordinated N1 and N3 atoms being Z, while the configurations about the other imine bonds are E.
Parameter | (I): M = CoII | (II): M = CuII | M—O1 | 1.8940 (17) | 1.8776 (14) | M—O2 | 1.8937 (17) | 1.8776 (14)a | M—N1 | 1.9988 (19) | 2.0211 (16) | M—N3 | 1.999 (2) | 2.0211 (16)a | N1—N2 | 1.411 (3) | 1.416 (2) | N3—N4 | 1.410 (3) | 1.416 (2)a | C7—N1 | 1.304 (3) | 1.294 (2) | C8—N2 | 1.251 (3) | 1.258 (3) | C21—N3 | 1.303 (3) | 1.294 (2)a | C22—N4 | 1.247 (3) | 1.258 (3)a | | | | O1—Co—O2 | 125.33 (8) | 180a | O1—Co—N1 | 94.06 (7) | 90.28 (6) | O1—Co—N3 | 112.12 (8) | 89.72 (6) | O2—Co—N1 | 113.82 (8) | 89.72 (6)a | O2—Co—N3 | 94.60 (8) | 90.28 (6)a | N1—Co—N3 | 119.03 (8) | 180a | Notes: (a) The CuII atom in (II) lies on a centre of inversion so O2 is equivalent to O1, N3 to N1, etc. and are related by the symmetry operation 1 − x, 1 − y, 1 − z. | |
| Figure 1 The molecular structure of (I) showing the atom-labelling scheme and displacement ellipsoids at the 35% probability level. |
Recently, the crystal structure of the precursor Schiff base ligand became available (Manawar, Gondaliya, Shah et al., 2019
). Here, each imine bond has an E-configuration and the bond lengths of the imine bonds, i.e. 1.281 (2) and 1.258 (3) Å, are intermediate to those noted in (I)
. A very similar conformation of the Schiff base ligand is found with a small twist about the central N—N bond [the C—N—N—C torsion angle = −172.7 (2)°]. The dihedral angles between the outer rings is 4.83 (13)°.
A distinct coordination geometry is found in the CuII complex, (II)
, Fig. 2
and Table 1
. The CuII atom lies on a crystallographic centre of inversion. As for (I)
, N,O-chelation is observed. From symmetry, the N2O2 donor set is strictly planar. The CuII atom lies 0.2582 (13) Å above the resultant square-plane. The chelate rings adopt an envelope conformation, as for (I)
, with the Cu atom lying 0.470 (2) Å above the plane through the remaining atoms of the chelate ring (r.m.s. deviation = 0.0129 Å). The magnitude of the dihedral angle between the five co-planar atoms of the chelate ring and the fused-benzene ring [1.43 (13)°] resembles the situation in (I)
, but that formed with pendent dichlorobenzene ring is quite distinct, at 82.63 (8)°, consistent with an orthogonal relationship. This is reflected in the C7—N1—N2—C8 torsion angle of 141.33 (19)°. The different configuration arises to avoid steric hindrance within the square-planar environment. The Cu—O,N bond lengths span a wider range, i.e. 0.14 Å, c.f. 0.11 Å for the Co—O,N bond lengths in (II)
, with the Cu—O bond lengths being shorter than the Co—O bonds, and the Cu—N bonds being longer than the Co—N bonds. Comparable trends are seen in the configurations of the imine bonds, Table 1
.
| Figure 2 The molecular structure of (II) showing the atom-labelling scheme and displacement ellipsoids at the 35% probability level. Unlabelled atoms are related by the symmetry operation 1 − x, 1 − y, 1 − z. |
3. Supramolecular features
The geometric parameters characterizing a number of the identified intermolecular contacts in the crystal of (I)
are listed in Table 2
. Globally, the molecular packing can be described as comprising inter-digitated layers stacked along the the b-axis direction. Thus, layers in the ac plane are consolidated by chlorobenzene-C—H⋯O(coordinated), chlorobenzene-C—H⋯π(fused-benzene ring) as well as π(fused-benzene, chlorobenzene)–π(chlorobenzene) interactions [Cg(C15–C20)⋯Cg(C23–C28) separation = 3.6460 (17) Å with angle of inclination = 5.57 (13)° for symmetry operation −1 + x, y, z and Cg(C23–C28)⋯Cg(C23–C28) = 3.6580 (16) Å with angle of inclination = 0° for symmetry operation 2 − x, 1 − y, 1 − z]; the specified π–π interactions involve rings derived from the O2-ligand only. A view of the supramolecular layer is shown in Fig. 3
(a). As highlighted in Fig. 3
(b), the layers inter-digitate along the b-axis. The connections between layers are chlorobenzene-C—H⋯π(fused-benzene ring) as well as π–π interactions (involving rings of the O1-ligand only) between centrosymmetrically related fused-benzene rings [π(C1–C6)⋯π(C1–C6) = 3.6916 (16) Å for symmetry operation 1 − x, − y, 1 − z and π(chlorobenzene)–π(chlorobenzene) rings = 3.7968 (19) Å for symmetry operation 1 − x, − y, 2 − z].
D—H⋯A | D—H | H⋯A | D⋯A | D—H⋯A | C27—H27⋯O1i | 0.93 | 2.35 | 3.114 (3) | 140 | C25—H25⋯Cg3ii | 0.93 | 2.86 | 3.647 (3) | 143 | Symmetry codes: (i) x+1, y, z; (ii) -x+2, -y+1, -z+1. | |
| Figure 3 Molecular packing in the crystal of (I) : (a) supramolecular layer sustained by C—H⋯O, C—H⋯π and π–π interactions shown as orange, blue and purple dashed lines, respectively, and (b) a view of the unit-cell contents in a projection down the c axis. |
The key feature of the molecular packing in the crystal of (II)
is the formation of π–π interactions between centrosymmetrically related molecules. To a first approximation, the molecular packing resembles that of (I)
in that layers assemble into a three-dimensional architecture. As seen in Fig. 4
(a), the layers are flat and are sustained by π(fused-benzene)–π(fused-benzene) [inter-centroid Cg(C1–C6)⋯Cg(C1—C6) separation = 3.8889 (15) Å for symmetry operation 1 − x, − y, 1 − z] and π(dichlorobenzene)—π(dichlorobenzene) [inter-centroid separation = Cg(C9–C14)⋯Cg(C9—C14) = 3.8889 (15) Å for symmetry operation − x, 1 − y, − z] interactions. The layers lie parallel to (10
) and stack without directional interactions between them. A view of the stacking of layers/unit-cell contents is shown in Fig. 4
(b).
| Figure 4 Molecular packing in the crystal of (II) : (a) supramolecular layer sustained by π–π interactions shown as purple dashed lines and (b) a view of the unit-cell contents in a projection down the b axis. |
4. Hirshfeld surface analysis
The Hirshfeld surfaces were calculated for each of (I)
and (II)
employing Crystal Explorer 17 (Turner et al., 2017
) and literature protocols (Tan et al., 2019
). This study was undertaken in order to determine the influence of weak, non-covalent interactions upon the molecular packing in the absence of conventional hydrogen bonding.
On the Hirshfeld surface mapped over dnorm for (I)
in Fig. 5
(a) and (b), the bright-red spots near the H27 atom of the (C23–C28) ring and the coordinating O1 atom are an indication of the C—H⋯O interaction. Referring to Table 3
, the presence of short interatomic contacts involving the CoII, chloride and chlorobenzene-hydrogen atoms and the atoms of the C1–C6 benzene ring are characterized as faint-red spots near the respective atoms on the dnorm-mapped Hirshfeld surface. The blue bump near the H25 atom and the bright-orange spot about the C1–C6 ring on the Hirshfeld surface mapped with shape-index property in Fig. 5
(c) correspond to the donor and acceptor of the C—H⋯π contact. The absence of strong, directional interactions in the crystal structure of (II)
is evident from its Hirshfeld surface mapped over dnorm in Fig. 6
, as the surface contains only some tiny, diffuse red spots near the atoms corresponding to short interatomic Cl⋯H and C⋯C contacts listed in Table 4
.
Contact | Distance | Symmetry operation | (I) | | | Cl1⋯Cl3 | 3.4993 (13) | x, −1 + y, z | Cl3⋯H7 | 2.70 | x, 1 + y, z | Cl4⋯H3 | 2.74 | 2 − x, − y, 1 − z | C1⋯H26 | 2.71 | 2 − x, 1 − y, 1 − z | C6⋯H26 | 2.76 | 2 − x, 1 − y, 1 − z | Co⋯C27 | 3.558 (3) | −1 + x, y, z | Co⋯H27 | 3.08 | −1 + x, y, z | H5⋯H12 | 2.23 | x, y, −1 + z | H5⋯H13 | 2.30 | x, y, −1 + z | (II) | | | Cl1⋯H7 | 2.81 | 1 − x, 1 − y, −z | C5⋯C7 | 3.378 (3) | 1 − x, −y, 1 − z | Cu⋯Cl2 | 3.2858 (7) | 1 + x, y, z | Notes: (a) The interatomic distances are calculated in Crystal Explorer 17 (Turner et al., 2017 ) whereby the X—H bond lengths are adjusted to their neutron values. | |
Contact | Percentage contribution | | (I) | (II) | H⋯H | 26.2 | 30.5 | O⋯H/H⋯O | 7.9 | 4.2 | C⋯H/H⋯C | 16.7 | 14.5 | Cl⋯H/H⋯Cl | 25.8 | 24.9 | C⋯C | 12.0 | 9.8 | N⋯H/H⋯ N | 5.5 | 6.2 | Cl⋯Cl | 2.2 | 0.4 | C⋯O/O⋯C | 0.5 | 0.3 | C⋯N/N⋯C | 0.5 | 1.3 | Cl⋯O/O⋯Cl | 0.2 | 0.4 | C⋯Cl/Cl⋯C | 1.9 | 3.9 | Cl⋯N/N⋯Cl | 0.2 | 1.6 | M⋯H/H⋯M | 0.4 | 0.1 | M⋯Cl/Cl⋯M | 0.0 | 1.9 | | |
| Figure 5 View of the Hirshfeld surface for (I) mapped (a) and (b) over dnorm in the range −0.123 to + 1.343 arbitrary units and (c) with the shape-index property highlighting intermolecular C—H⋯π/π⋯H—C contacts. |
| Figure 6 A view of the Hirshfeld surface for (II) mapped over dnorm in the range −0.016 to 1.528 arbitrary units. |
On the Hirshfeld surfaces mapped over the electrostatic potential for (I)
in Fig. 7
(a), the donors and acceptors of the C—H⋯O and C—H⋯π contacts (Table 3
) are viewed as blue and red regions near the respective atoms corresponding to positive and negative electrostatic potentials. The presence of a blue region near the CuII atom and red region near the Cl2 atom in the corresponding surface for (II)
in Fig. 7
(b) is an indication of a short intermolecular Cu⋯Cl2 interaction [3.2858 (7) Å], as discussed further below, see Computational chemistry. The influence of π–π stacking interactions in each of the crystals of (I)
and (II)
is evident as the flat regions about the participating aromatic rings on the Hirshfeld surfaces mapped over curvedness illustrated in Fig. 8
(a)–(d).
| Figure 7 Views of the Hirshfeld surfaces mapped over the electrostatic potential (the red and blue regions represent negative and positive electrostatic potentials, respectively) for (a) (I) in the range −0.084 to +0.061 atomic units and (b) (II) in the range −0.095 to +0.163 atomic units. |
| Figure 8 Views of Hirshfeld surfaces mapped over curvedness for (a) and (b) (I) , and (c) and (d) for (II) . The flat regions about aromatic constituents labelled with Cg(1)–Cg(4) for (I) , and Cg(1) and Cg(2) for (II) indicate the involvement of these rings in π–π stacking interactions |
Given the different coordination geometries in (I)
and (II)
, it was thought of interest to calculate the Hirshfeld surfaces about the individual metal centres (Pinto et al., 2019
). The different coordination geometries, approximately trigonal pyramidal for CoII, Fig. 9
(a) and (b), and square-planar for CuII in Fig. 9
(c) and (d), are clearly evident from the illustrated surfaces although the M—O and M—N bond lengths are similar, at least to a first approximation, in (I)
and (II)
, Table 1
.
| Figure 9 Views of the Hirshfeld surfaces calculated for the CoII (I) and CuII (II) centres alone, highlighting the coordination geometries formed by the N2O2 donor sets mapped over (a) the distance de external to the surface in the range 0.922 to 2.221 Å for (I) , (b) the shape-index (S) from −1.0 to +1.0 (arbitrary units) for (I) , (c) the distance de external to the surface in the range 0.919 to 2.114 Å for (II) and (d) the shape-index (S) from −1.0 to +1.0 (arbitrary units) for (II) . |
The different coordination geometries about the metal centres are also reflected in the two-dimensional fingerprint plots shown in Fig. 10
, only taking into account the Hirshfeld surface about the metal atom. The distribution of aligned red points from de + di ∼1.8 Å (lower portion) and de + di ∼2.0 Å (upper portion) for the Co—O and Co—N bonds, respectively, in (I)
show different inclinations, Fig. 10
(a), whereas the superimposed red points in the case of (II)
, Fig. 10
(b), arise as a result of the symmetrical coordination geometry. For (I)
, the presence of short intramolecular Co⋯H contacts formed with the chlorobenzene-H8 and H22 atoms (Co⋯H8 = 2.64 Å and Co⋯H22 = 2.55 Å) result in dissymmetry in the Hirshfeld surface and are characterized as bright-orange spots on the shape-index-mapped surface in Fig. 9
(a). The square-planar coordination geometry formed by the N2O2 donor set in (II)
results in an approximate cuboid Hirshfeld surface with rounded corners and edges.
| Figure 10 The two-dimensional fingerprint plots taking into account only the Hirshfeld surface about the metal centre in (a) (I) and (b) (II) . |
The overall two-dimensional fingerprint plots for (I)
and (II)
are shown in Fig. 11
(a), and those delineated into H⋯H, O⋯H/H⋯O, Cl⋯H/H⋯Cl, C⋯H/H⋯C and C⋯C contacts are illustrated in Fig. 11
(b)–11(f), respectively. The percentage contributions from different interatomic contacts to the Hirshfeld surfaces of (I)
and (II)
are summarized in Table 4
. The presence of chloride in both crystals, and their participation in intermolecular contacts, has decreased the percentage contributions from H⋯H contacts to the respective Hirshfeld surfaces, Table 4
.
| Figure 11 (a) A comparison of the full two-dimensional fingerprint plot for each of (I) and (II) and those delineated into (b) H⋯H, (c) O⋯H/H⋯O, (d) Cl⋯H/H⋯Cl, (e) C⋯H/H⋯C and (f) C⋯C contacts. |
In the fingerprint plot delineated into H⋯H contacts of Fig. 11
(b), the short interatomic contacts result in a peak at de + di ∼2.3 Å in the crystal of (I)
while H⋯H in (II)
are at van der Waals separations or longer. The presence of the C—H⋯O contact in (I)
is recognized as the pair of spikes at de + di ∼2.2 Å in the fingerprint plot delineated into O⋯H/H⋯O contacts of Fig. 11
(c) whereas the comparatively small contribution from these contacts in (II)
, Table 4
, show the points farther than sum of their van der Waals radii. The pair of forceps-like tips at de + di ∼2.8 Å in the fingerprint plots delineated into Cl⋯H/H⋯Cl contacts in Fig. 11
(d) for each of (I)
and (II)
reflect the presence of Cl⋯H contacts in their crystals; for (II)
, these are beyond the sum of the van der Waals radii. From the fingerprint plot delineated into C⋯H/H⋯C contacts, Fig. 11
(e), the pair of short tips at de + di ∼2.7 Å indicate the presence of C⋯H and C—H⋯π contacts in (I)
, by contrast to only van der Waals contacts in (II)
. In the fingerprint plot delineated into C⋯C contacts for (I)
and (II)
, Fig. 11
(f), the influence of π–π stacking interactions are characterized as the distribution of green points in the plot around de = di = 1.8 Å.
Referring to Fig. 12
(a), the distribution of points in the form of a thin line from de + di ∼3.7 Å in the fingerprint plot delineated into Cl⋯Cl contacts for (I)
is an indication of influence of these contacts on the packing of (I)
; this is confirmed in the next section, Computational study. The fingerprint plot delineated into Cu⋯Cl/Cl⋯Cu contacts of Fig. 12
(b), with the small, i.e. 1.9%, but important contribution to the Hirshfeld surface of (II)
is the result of a Cu⋯Cl interaction prominent in its molecular packing, as justified from the interaction energy calculations described in the next section.
| Figure 12 The fingerprint plot delineated into (a) Cl⋯Cl contacts for (I) and (b) Cu⋯Cl/Cl⋯Cu contacts for (II) . |
5. Computational chemistry
The pairwise interaction energies between the molecules in the crystals of each of (I)
and (II)
were calculated by summing up four energy components, comprising electrostatic (Eele), polarization (Epol), dispersion (Edis) and exchange-repulsion (Erep) as per the literature (Turner et al., 2017
). In the present study, the energies were obtained by using the wave function calculated at the HF/3-21G level of theory. The nature and the strength of the energies for the key identified intermolecular interactions are quantitatively summarized in Tables 5
and 6
for (I)
and (II)
, respectively.
Contact | R (Å) | Eele | Epol | Edis | Erep | Etot | H27⋯Coi + | 8.81 | −21.7 | −6.7 | −71.3 | 41.6 | −57.0 | C27⋯Coi + | | | | | | | C27—H27⋯O1i + | | | | | | | Cg(C15–C20)⋯Cg(C23–C28)i | | | | | | | Cg(C9–C14) ⋯Cg(C9–C14)ii | 9.60 | −29.6 | −7.6 | −71.9 | 32.5 | −73.5 | Cg(C1–C6) ⋯Cg(C1–C6)iii | 10.19 | −23.4 | −5.5 | −58.3 | 29.4 | −56.0 | Cl4⋯H3iv + | 10.54 | −12.7 | −1.2 | −26.4 | 19.8 | −21.4 | Cl1⋯Cl3iv | | | | | | | Cl3⋯H7v | 10.48 | −3.9 | −1.3 | −14.8 | 13.4 | −7.3 | C1⋯H26vi + | 9.86 | −37.0 | −8.0 | −84.1 | 48.4 | −79.5 | C6⋯H26vi + | | | | | | | C25–H25⋯Cg(C1–C6)vi + | | | | | | | Cg(C23–C28)⋯Cg(C23–C28)vi | | | | | | | Symmetry codes: (i) 1 + x, y, z; (ii) 1 − x, − y, 2 − z; (iii) 1 − x, − y, 1 − z; (iv) 2 − x, − y, 1 − z; (v) x, 1 + y, z; (vi) 2 − x, 1 − y, 1 − z. | |
Contact | R (Å) | Eele | Epol | Edis | Erep | Etot | Cg(C9–C14)⋯Cg(C9–C14)i | 12.94 | −0.7 | −3.0 | −44.5 | 14.8 | −30.8 | Cu⋯Cl2ii | 8.13 | −33.0 | −5.6 | −64.1 | 44.4 | −59.0 | Cl1⋯H7iii | 9.74 | −3.7 | −2.5 | −25.7 | 15.4 | −16.0 | C5⋯C7iv + | 8.51 | −14.4 | −4.7 | −68.5 | 36.8 | −49.6 | Cg(C1–C6)⋯Cg(C1–C6)iv | | | | | | | Symmetry codes: (i) − x, 1 − y, − z; (ii) 1 + x, y, z; (iii) 1 − x, 1 − y, −z; (iv) 1 − x, − y, 1 − z. | |
For (I)
, among the intermolecular energies listed in Table 5
, the atoms of (C23–C28) ring involved in the short interatomic C⋯H/H⋯C contacts, intermolecular C—H⋯π and π–π stacking interactions between the same pair of symmetry-related molecules have maximum interaction energies. The dispersive component makes a major contribution to all of the intermolecular interactions in the crystal of (I)
. The low interaction energies for Cl⋯H and Cl⋯Cl contacts are consistent with the small contributions from these contacts in the crystal. The presence of a Cu⋯Cl2 contact in the crystal of (II)
is an important feature of the packing. This interaction shows maximum interaction energy (Table 6
) with significant contributions from the electrostatic component compared to π–π stacking and other intermolecular interactions influential in the molecular packing.
The magnitudes of intermolecular energies are represented graphically in the energy framework diagrams of Fig. 13
(a)–(f). Here, the supramolecular architecture of each crystal is visualized through cylinders joining the centroids of molecular pairs using a red, green and blue colour scheme, representing the Eele, Edisp and Etot components, respectively; the stronger the interaction, the thicker the cylinder.
| Figure 13 The energy frameworks calculated for (I) showing the (a) electrostatic potential force, (b) dispersion force and (c) total energy; the equivalent diagrams for (II) are shown in (d)–(f). The energy frameworks were adjusted to the same to same scale factor of 30 with a cut-off value of 3 kJ mol−1 within 2 × 2 × 2 unit cells. |
6. Database survey
Schiff bases related to those reported in (I)
and (II)
, i.e. having two imine functionalities and a single phenol/phenoxide atom/ion on one ring only are quite rare. Thus, the only structure of an analogue available for comparison is a N,O-chelated dimethylaluminium compound with the ring bearing the phenoxide-oxygen also carrying t-butyl groups at the 3,5 positions and the second benzene ring bearing a chlorine atom in the 4-position (UPEKEH; Hsu et al., 2017
). By contrast, there are numerous examples of coordination complexes derived from Schiff base molecules with two 2-phenol substituents in each ring, LH2. In these instances, the dinegative Schiff base anion N,O-chelates two metal centres such as in binuclear Co2(L1)3 (JUKZOG; Liu et al., 2015
), with a fac-N3O3 donor set within an octahedral geometry, and Cu2(L2)3(PPh3)2 (VOWBAM; Prakash et al., 2015
) with tetrahedral NOP2 donor sets; for the L1 dianion, there are 3-ethoxy substituents in each ring and for L2, the are 4-chloro substituents.
8. Refinement
Crystal data, data collection and structure refinement details are summarized in Table 7
. The carbon-bound H atoms were placed in calculated positions (C—H = 0.93 Å) and were included in the refinement in the riding model approximation, with Uiso(H) set to 1.2Ueq(C).
| (I) | (II) | Crystal data | Chemical formula | [Co(C14H9Cl2N2O)2] | [Cu(C14H9Cl2N2O)2] | Mr | 643.19 | 647.80 | Crystal system, space group | Triclinic, P![[\overline{1}]](teximages/hb7872fi1.gif) | Triclinic, P![[\overline{1}]](teximages/hb7872fi1.gif) | Temperature (K) | 296 | 296 | a, b, c (Å) | 8.8137 (10), 10.4801 (12), 15.0785 (17) | 8.1300 (7), 8.5072 (11), 9.7386 (13) | α, β, γ (°) | 85.684 (7), 77.984 (7), 84.965 (7) | 83.240 (4), 87.646 (3), 81.533 (4) | V (Å3) | 1354.7 (3) | 661.39 (14) | Z | 2 | 1 | Radiation type | Mo Kα | Mo Kα | μ (mm−1) | 1.06 | 1.27 | Crystal size (mm) | 0.35 × 0.30 × 0.30 | 0.35 × 0.35 × 0.30 | | Data collection | Diffractometer | Bruker Kappa APEXII CCD | Bruker Kappa APEXII CCD | Absorption correction | Multi-scan (SADABS; Bruker, 2004 ) | Multi-scan (SADABS; Bruker, 2004 ) | Tmin, Tmax | 0.631, 0.746 | 0.637, 0.714 | No. of measured, independent and observed [I > 2σ(I)] reflections | 45690, 6959, 4590 | 5554, 3090, 2708 | Rint | 0.102 | 0.021 | (sin θ/λ)max (Å−1) | 0.678 | 0.667 | | Refinement | R[F2 > 2σ(F2)], wR(F2), S | 0.045, 0.112, 1.04 | 0.033, 0.093, 1.05 | No. of reflections | 6959 | 3090 | No. of parameters | 352 | 178 | H-atom treatment | H-atom parameters constrained | H-atom parameters constrained | Δρmax, Δρmin (e Å−3) | 0.45, −0.50 | 0.44, −0.46 | Computer programs: APEX2 and SAINT (Bruker, 2004 ),SIR92 (Altomare et al., 1994 ), SHELXL2017/1 (Sheldrick, 2015 ), ORTEP-3 for Windows (Farrugia, 2012 ), DIAMOND (Brandenburg, 2006 ) and publCIF (Westrip, 2010 ). | |
Supporting information
For both structures, data collection: APEX2 (Bruker, 2004); cell refinement: APEX2/SAINT (Bruker, 2004); data reduction: SAINT (Bruker, 2004); program(s) used to solve structure: SIR92 (Altomare et al., 1994); program(s) used to refine structure: SHELXL2017/1 (Sheldrick, 2015); molecular graphics: ORTEP-3 for Windows (Farrugia, 2012), DIAMOND (Brandenburg, 2006); software used to prepare material for publication: publCIF (Westrip, 2010).
Bis(2-{[(2,6-dichlorobenzylidene)hydrazinylidene]methyl}phenolato)cobalt(II) (I)
top Crystal data top [Co(C14H9Cl2N2O)2] | Z = 2 |
Mr = 643.19 | F(000) = 650 |
Triclinic, P1 | Dx = 1.577 Mg m−3 |
a = 8.8137 (10) Å | Mo Kα radiation, λ = 0.71073 Å |
b = 10.4801 (12) Å | Cell parameters from 5585 reflections |
c = 15.0785 (17) Å | θ = 2.3–22.4° |
α = 85.684 (7)° | µ = 1.06 mm−1 |
β = 77.984 (7)° | T = 296 K |
γ = 84.965 (7)° | Block, red |
V = 1354.7 (3) Å3 | 0.35 × 0.30 × 0.30 mm |
Data collection top Bruker Kappa APEXII CCD diffractometer | 4590 reflections with I > 2σ(I) |
Radiation source: X-ray tube | Rint = 0.102 |
ω and φ scan | θmax = 28.8°, θmin = 1.4° |
Absorption correction: multi-scan (SADABS; Bruker, 2004) | h = −11→11 |
Tmin = 0.631, Tmax = 0.746 | k = −14→14 |
45690 measured reflections | l = −20→20 |
6959 independent reflections | |
Refinement top Refinement on F2 | Primary atom site location: structure-invariant direct methods |
Least-squares matrix: full | Secondary atom site location: difference Fourier map |
R[F2 > 2σ(F2)] = 0.045 | Hydrogen site location: inferred from neighbouring sites |
wR(F2) = 0.112 | H-atom parameters constrained |
S = 1.04 | w = 1/[σ2(Fo2) + (0.031P)2 + 0.0591P] where P = (Fo2 + 2Fc2)/3 |
6959 reflections | (Δ/σ)max = 0.001 |
352 parameters | Δρmax = 0.45 e Å−3 |
0 restraints | Δρmin = −0.50 e Å−3 |
Special details top Geometry. All esds (except the esd in the dihedral angle between two l.s. planes) are estimated using the full covariance matrix. The cell esds are taken into account individually in the estimation of esds in distances, angles and torsion angles; correlations between esds in cell parameters are only used when they are defined by crystal symmetry. An approximate (isotropic) treatment of cell esds is used for estimating esds involving l.s. planes. |
Fractional atomic coordinates and isotropic or equivalent isotropic displacement parameters (Å2) top | x | y | z | Uiso*/Ueq | |
Co | 0.44734 (4) | 0.27310 (3) | 0.68541 (2) | 0.03428 (11) | |
Cl1 | 0.88398 (11) | −0.08192 (9) | 0.85762 (6) | 0.0821 (3) | |
Cl2 | 0.45007 (10) | 0.29954 (8) | 0.98752 (6) | 0.0675 (2) | |
Cl3 | 0.79790 (9) | 0.73174 (7) | 0.69395 (6) | 0.0643 (2) | |
Cl4 | 0.96728 (8) | 0.23680 (7) | 0.60664 (6) | 0.0566 (2) | |
O1 | 0.48493 (19) | 0.28424 (16) | 0.55684 (12) | 0.0386 (4) | |
O2 | 0.25211 (19) | 0.24964 (17) | 0.76315 (12) | 0.0441 (4) | |
N1 | 0.6051 (2) | 0.12543 (18) | 0.69189 (13) | 0.0310 (4) | |
N2 | 0.6554 (2) | 0.0714 (2) | 0.77036 (14) | 0.0411 (5) | |
N3 | 0.4754 (2) | 0.43864 (19) | 0.73568 (14) | 0.0352 (5) | |
N4 | 0.6127 (2) | 0.5040 (2) | 0.71934 (17) | 0.0470 (6) | |
C1 | 0.5601 (3) | 0.1984 (2) | 0.50173 (16) | 0.0320 (5) | |
C2 | 0.6504 (3) | 0.0906 (2) | 0.53011 (16) | 0.0328 (5) | |
C3 | 0.7307 (3) | 0.0055 (3) | 0.46435 (18) | 0.0424 (6) | |
H3 | 0.788970 | −0.065835 | 0.483233 | 0.051* | |
C4 | 0.7258 (3) | 0.0241 (3) | 0.37463 (19) | 0.0496 (7) | |
H4 | 0.780924 | −0.032128 | 0.332457 | 0.060* | |
C5 | 0.6353 (3) | 0.1305 (3) | 0.34809 (19) | 0.0488 (7) | |
H5 | 0.629642 | 0.144282 | 0.287166 | 0.059* | |
C6 | 0.5546 (3) | 0.2148 (3) | 0.40882 (17) | 0.0403 (6) | |
H6 | 0.495089 | 0.284277 | 0.388505 | 0.048* | |
C7 | 0.6684 (3) | 0.0616 (2) | 0.62082 (17) | 0.0355 (6) | |
H7 | 0.732456 | −0.011148 | 0.630792 | 0.043* | |
C8 | 0.6244 (3) | 0.1407 (3) | 0.83673 (17) | 0.0394 (6) | |
H8 | 0.574799 | 0.221594 | 0.828883 | 0.047* | |
C9 | 0.6609 (3) | 0.1035 (2) | 0.92574 (17) | 0.0377 (6) | |
C10 | 0.7686 (3) | 0.0031 (3) | 0.94344 (19) | 0.0467 (7) | |
C11 | 0.7896 (4) | −0.0315 (3) | 1.0308 (2) | 0.0573 (8) | |
H11 | 0.860799 | −0.099067 | 1.041003 | 0.069* | |
C12 | 0.7048 (4) | 0.0344 (4) | 1.1022 (2) | 0.0646 (9) | |
H12 | 0.717869 | 0.010093 | 1.160909 | 0.078* | |
C13 | 0.6007 (4) | 0.1359 (3) | 1.0883 (2) | 0.0588 (8) | |
H13 | 0.544591 | 0.180895 | 1.136912 | 0.071* | |
C14 | 0.5809 (3) | 0.1697 (3) | 1.00133 (19) | 0.0448 (7) | |
C15 | 0.1585 (3) | 0.3409 (3) | 0.80467 (17) | 0.0397 (6) | |
C16 | 0.2042 (3) | 0.4638 (3) | 0.81575 (18) | 0.0410 (6) | |
C17 | 0.0932 (3) | 0.5554 (3) | 0.8613 (2) | 0.0575 (8) | |
H17 | 0.123982 | 0.635619 | 0.869447 | 0.069* | |
C18 | −0.0578 (4) | 0.5289 (4) | 0.8936 (2) | 0.0671 (10) | |
H18 | −0.130212 | 0.591163 | 0.921242 | 0.081* | |
C19 | −0.1010 (3) | 0.4085 (4) | 0.8846 (2) | 0.0649 (9) | |
H19 | −0.203286 | 0.389479 | 0.907762 | 0.078* | |
C20 | 0.0023 (3) | 0.3155 (3) | 0.84240 (19) | 0.0523 (8) | |
H20 | −0.030665 | 0.234560 | 0.838523 | 0.063* | |
C21 | 0.3586 (3) | 0.5031 (3) | 0.78469 (19) | 0.0440 (7) | |
H21 | 0.377708 | 0.582955 | 0.801210 | 0.053* | |
C22 | 0.7287 (3) | 0.4444 (2) | 0.67455 (18) | 0.0374 (6) | |
H22 | 0.712804 | 0.364115 | 0.657169 | 0.045* | |
C23 | 0.8864 (3) | 0.4856 (2) | 0.64614 (16) | 0.0333 (5) | |
C24 | 0.9300 (3) | 0.6101 (2) | 0.64938 (19) | 0.0414 (6) | |
C25 | 1.0819 (3) | 0.6408 (3) | 0.6154 (2) | 0.0511 (7) | |
H25 | 1.108921 | 0.724006 | 0.618188 | 0.061* | |
C26 | 1.1928 (3) | 0.5492 (3) | 0.5775 (2) | 0.0525 (8) | |
H26 | 1.293927 | 0.571199 | 0.553932 | 0.063* | |
C27 | 1.1554 (3) | 0.4260 (3) | 0.57441 (18) | 0.0453 (7) | |
H27 | 1.230392 | 0.363795 | 0.549057 | 0.054* | |
C28 | 1.0048 (3) | 0.3955 (3) | 0.60946 (18) | 0.0384 (6) | |
Atomic displacement parameters (Å2) top | U11 | U22 | U33 | U12 | U13 | U23 |
Co | 0.03317 (18) | 0.0346 (2) | 0.0336 (2) | 0.00280 (14) | −0.00453 (14) | −0.00479 (15) |
Cl1 | 0.1017 (7) | 0.0828 (6) | 0.0679 (6) | 0.0502 (5) | −0.0448 (5) | −0.0328 (5) |
Cl2 | 0.0717 (5) | 0.0700 (6) | 0.0533 (5) | 0.0181 (4) | −0.0018 (4) | −0.0142 (4) |
Cl3 | 0.0601 (5) | 0.0356 (4) | 0.1007 (7) | 0.0009 (3) | −0.0221 (4) | −0.0150 (4) |
Cl4 | 0.0460 (4) | 0.0380 (4) | 0.0810 (6) | 0.0041 (3) | −0.0014 (4) | −0.0129 (4) |
O1 | 0.0414 (9) | 0.0376 (10) | 0.0348 (10) | 0.0096 (8) | −0.0074 (8) | −0.0044 (8) |
O2 | 0.0387 (9) | 0.0430 (11) | 0.0459 (12) | −0.0004 (8) | 0.0022 (8) | −0.0058 (9) |
N1 | 0.0356 (10) | 0.0300 (11) | 0.0274 (11) | −0.0004 (8) | −0.0075 (9) | −0.0003 (9) |
N2 | 0.0546 (13) | 0.0363 (13) | 0.0329 (13) | 0.0050 (10) | −0.0137 (10) | −0.0005 (10) |
N3 | 0.0302 (10) | 0.0345 (12) | 0.0393 (13) | 0.0028 (9) | −0.0046 (9) | −0.0045 (10) |
N4 | 0.0381 (12) | 0.0387 (13) | 0.0618 (16) | −0.0019 (10) | −0.0005 (11) | −0.0162 (12) |
C1 | 0.0331 (12) | 0.0312 (13) | 0.0321 (14) | −0.0049 (10) | −0.0069 (10) | −0.0014 (11) |
C2 | 0.0374 (13) | 0.0315 (13) | 0.0303 (13) | −0.0020 (10) | −0.0076 (10) | −0.0059 (11) |
C3 | 0.0452 (15) | 0.0403 (16) | 0.0414 (16) | 0.0036 (12) | −0.0086 (12) | −0.0087 (13) |
C4 | 0.0596 (17) | 0.0513 (18) | 0.0366 (16) | 0.0003 (14) | −0.0036 (13) | −0.0166 (14) |
C5 | 0.0617 (18) | 0.0576 (19) | 0.0302 (15) | −0.0106 (15) | −0.0126 (13) | −0.0062 (14) |
C6 | 0.0453 (14) | 0.0424 (16) | 0.0347 (15) | −0.0019 (12) | −0.0131 (12) | −0.0010 (12) |
C7 | 0.0407 (13) | 0.0293 (13) | 0.0357 (15) | 0.0045 (10) | −0.0085 (11) | −0.0031 (11) |
C8 | 0.0463 (14) | 0.0368 (15) | 0.0349 (15) | 0.0065 (12) | −0.0112 (12) | −0.0034 (12) |
C9 | 0.0461 (14) | 0.0375 (15) | 0.0306 (14) | −0.0059 (12) | −0.0096 (11) | −0.0010 (12) |
C10 | 0.0574 (17) | 0.0446 (17) | 0.0423 (17) | 0.0006 (13) | −0.0195 (14) | −0.0087 (13) |
C11 | 0.076 (2) | 0.0532 (19) | 0.0510 (19) | −0.0012 (16) | −0.0361 (17) | 0.0022 (16) |
C12 | 0.083 (2) | 0.079 (2) | 0.0371 (18) | −0.015 (2) | −0.0227 (17) | 0.0032 (17) |
C13 | 0.074 (2) | 0.070 (2) | 0.0328 (17) | −0.0076 (18) | −0.0091 (15) | −0.0092 (16) |
C14 | 0.0535 (16) | 0.0464 (17) | 0.0349 (15) | −0.0098 (13) | −0.0069 (12) | −0.0034 (13) |
C15 | 0.0344 (13) | 0.0524 (17) | 0.0295 (14) | −0.0004 (12) | −0.0033 (11) | 0.0037 (12) |
C16 | 0.0372 (13) | 0.0466 (16) | 0.0343 (15) | 0.0073 (12) | 0.0004 (11) | −0.0030 (12) |
C17 | 0.0498 (17) | 0.0564 (19) | 0.056 (2) | 0.0133 (14) | 0.0064 (14) | −0.0076 (16) |
C18 | 0.0467 (17) | 0.076 (3) | 0.065 (2) | 0.0186 (17) | 0.0105 (15) | −0.0072 (19) |
C19 | 0.0350 (15) | 0.097 (3) | 0.055 (2) | 0.0029 (17) | 0.0038 (14) | 0.0023 (19) |
C20 | 0.0389 (15) | 0.064 (2) | 0.0497 (19) | −0.0041 (14) | −0.0014 (13) | 0.0016 (15) |
C21 | 0.0416 (14) | 0.0365 (15) | 0.0509 (18) | 0.0043 (12) | −0.0035 (12) | −0.0081 (13) |
C22 | 0.0348 (13) | 0.0298 (14) | 0.0482 (16) | 0.0003 (10) | −0.0097 (11) | −0.0057 (12) |
C23 | 0.0348 (12) | 0.0344 (14) | 0.0317 (14) | −0.0014 (10) | −0.0110 (10) | 0.0014 (11) |
C24 | 0.0452 (15) | 0.0350 (15) | 0.0491 (17) | −0.0046 (11) | −0.0215 (13) | 0.0001 (12) |
C25 | 0.0534 (17) | 0.0454 (17) | 0.061 (2) | −0.0170 (14) | −0.0232 (15) | 0.0048 (15) |
C26 | 0.0381 (15) | 0.068 (2) | 0.0531 (19) | −0.0158 (14) | −0.0116 (13) | 0.0036 (16) |
C27 | 0.0345 (13) | 0.0602 (19) | 0.0405 (16) | 0.0005 (13) | −0.0077 (12) | −0.0025 (14) |
C28 | 0.0365 (13) | 0.0405 (15) | 0.0387 (15) | −0.0001 (11) | −0.0114 (11) | 0.0014 (12) |
Geometric parameters (Å, º) top Co—O2 | 1.8937 (17) | C9—C14 | 1.406 (4) |
Co—O1 | 1.8940 (17) | C10—C11 | 1.385 (4) |
Co—N1 | 1.9988 (19) | C11—C12 | 1.371 (4) |
Co—N3 | 1.999 (2) | C11—H11 | 0.9300 |
Cl1—C10 | 1.723 (3) | C12—C13 | 1.376 (4) |
Cl2—C14 | 1.733 (3) | C12—H12 | 0.9300 |
Cl3—C24 | 1.725 (3) | C13—C14 | 1.375 (4) |
Cl4—C28 | 1.729 (3) | C13—H13 | 0.9300 |
O1—C1 | 1.310 (3) | C15—C20 | 1.416 (3) |
O2—C15 | 1.313 (3) | C15—C16 | 1.414 (4) |
N1—C7 | 1.304 (3) | C16—C17 | 1.416 (4) |
N1—N2 | 1.411 (3) | C16—C21 | 1.431 (3) |
N2—C8 | 1.251 (3) | C17—C18 | 1.363 (4) |
N3—C21 | 1.303 (3) | C17—H17 | 0.9300 |
N3—N4 | 1.410 (3) | C18—C19 | 1.374 (5) |
N4—C22 | 1.247 (3) | C18—H18 | 0.9300 |
C1—C6 | 1.409 (3) | C19—C20 | 1.371 (4) |
C1—C2 | 1.416 (3) | C19—H19 | 0.9300 |
C2—C3 | 1.417 (3) | C20—H20 | 0.9300 |
C2—C7 | 1.417 (3) | C21—H21 | 0.9300 |
C3—C4 | 1.361 (4) | C22—C23 | 1.461 (3) |
C3—H3 | 0.9300 | C22—H22 | 0.9300 |
C4—C5 | 1.396 (4) | C23—C28 | 1.397 (3) |
C4—H4 | 0.9300 | C23—C24 | 1.399 (3) |
C5—C6 | 1.366 (4) | C24—C25 | 1.388 (4) |
C5—H5 | 0.9300 | C25—C26 | 1.374 (4) |
C6—H6 | 0.9300 | C25—H25 | 0.9300 |
C7—H7 | 0.9300 | C26—C27 | 1.367 (4) |
C8—C9 | 1.461 (3) | C26—H26 | 0.9300 |
C8—H8 | 0.9300 | C27—C28 | 1.379 (3) |
C9—C10 | 1.402 (4) | C27—H27 | 0.9300 |
| | | |
O2—Co—O1 | 125.33 (8) | C11—C12—H12 | 119.5 |
O2—Co—N1 | 113.82 (8) | C13—C12—H12 | 119.5 |
O1—Co—N1 | 94.06 (7) | C14—C13—C12 | 119.0 (3) |
O2—Co—N3 | 94.60 (8) | C14—C13—H13 | 120.5 |
O1—Co—N3 | 112.12 (8) | C12—C13—H13 | 120.5 |
N1—Co—N3 | 119.03 (8) | C13—C14—C9 | 122.7 (3) |
C1—O1—Co | 127.34 (15) | C13—C14—Cl2 | 117.0 (2) |
C15—O2—Co | 125.37 (16) | C9—C14—Cl2 | 120.3 (2) |
C7—N1—N2 | 111.4 (2) | O2—C15—C20 | 118.7 (3) |
C7—N1—Co | 121.70 (16) | O2—C15—C16 | 124.1 (2) |
N2—N1—Co | 126.78 (15) | C20—C15—C16 | 117.2 (2) |
C8—N2—N1 | 114.8 (2) | C15—C16—C17 | 119.4 (2) |
C21—N3—N4 | 112.1 (2) | C15—C16—C21 | 124.2 (2) |
C21—N3—Co | 121.09 (17) | C17—C16—C21 | 116.4 (3) |
N4—N3—Co | 126.71 (15) | C18—C17—C16 | 121.6 (3) |
C22—N4—N3 | 114.3 (2) | C18—C17—H17 | 119.2 |
O1—C1—C6 | 118.8 (2) | C16—C17—H17 | 119.2 |
O1—C1—C2 | 123.5 (2) | C17—C18—C19 | 118.9 (3) |
C6—C1—C2 | 117.7 (2) | C17—C18—H18 | 120.6 |
C3—C2—C7 | 116.8 (2) | C19—C18—H18 | 120.6 |
C3—C2—C1 | 118.9 (2) | C18—C19—C20 | 121.9 (3) |
C7—C2—C1 | 124.4 (2) | C18—C19—H19 | 119.0 |
C4—C3—C2 | 122.6 (3) | C20—C19—H19 | 119.0 |
C4—C3—H3 | 118.7 | C19—C20—C15 | 120.9 (3) |
C2—C3—H3 | 118.7 | C19—C20—H20 | 119.5 |
C3—C4—C5 | 117.7 (3) | C15—C20—H20 | 119.5 |
C3—C4—H4 | 121.1 | N3—C21—C16 | 126.8 (3) |
C5—C4—H4 | 121.1 | N3—C21—H21 | 116.6 |
C6—C5—C4 | 122.1 (3) | C16—C21—H21 | 116.6 |
C6—C5—H5 | 119.0 | N4—C22—C23 | 127.7 (2) |
C4—C5—H5 | 119.0 | N4—C22—H22 | 116.1 |
C5—C6—C1 | 121.1 (3) | C23—C22—H22 | 116.1 |
C5—C6—H6 | 119.5 | C28—C23—C24 | 116.2 (2) |
C1—C6—H6 | 119.5 | C28—C23—C22 | 118.2 (2) |
N1—C7—C2 | 127.3 (2) | C24—C23—C22 | 125.6 (2) |
N1—C7—H7 | 116.4 | C25—C24—C23 | 120.9 (3) |
C2—C7—H7 | 116.4 | C25—C24—Cl3 | 117.3 (2) |
N2—C8—C9 | 124.5 (2) | C23—C24—Cl3 | 121.8 (2) |
N2—C8—H8 | 117.7 | C26—C25—C24 | 120.5 (3) |
C9—C8—H8 | 117.7 | C26—C25—H25 | 119.7 |
C10—C9—C14 | 116.0 (2) | C24—C25—H25 | 119.7 |
C10—C9—C8 | 125.2 (2) | C27—C26—C25 | 120.4 (3) |
C14—C9—C8 | 118.8 (2) | C27—C26—H26 | 119.8 |
C11—C10—C9 | 121.7 (3) | C25—C26—H26 | 119.8 |
C11—C10—Cl1 | 116.5 (2) | C26—C27—C28 | 118.9 (3) |
C9—C10—Cl1 | 121.8 (2) | C26—C27—H27 | 120.5 |
C12—C11—C10 | 119.7 (3) | C28—C27—H27 | 120.5 |
C12—C11—H11 | 120.2 | C27—C28—C23 | 123.1 (2) |
C10—C11—H11 | 120.2 | C27—C28—Cl4 | 116.4 (2) |
C11—C12—C13 | 121.0 (3) | C23—C28—Cl4 | 120.48 (19) |
| | | |
O2—Co—O1—C1 | −108.33 (19) | C12—C13—C14—Cl2 | −179.2 (2) |
N1—Co—O1—C1 | 14.8 (2) | C10—C9—C14—C13 | −2.7 (4) |
N3—Co—O1—C1 | 138.45 (19) | C8—C9—C14—C13 | 175.8 (3) |
O1—Co—O2—C15 | −99.8 (2) | C10—C9—C14—Cl2 | 177.6 (2) |
N1—Co—O2—C15 | 146.13 (19) | C8—C9—C14—Cl2 | −3.9 (3) |
N3—Co—O2—C15 | 21.5 (2) | Co—O2—C15—C20 | 164.92 (18) |
C7—N1—N2—C8 | 166.6 (2) | Co—O2—C15—C16 | −16.2 (4) |
Co—N1—N2—C8 | −17.3 (3) | O2—C15—C16—C17 | 179.6 (3) |
C21—N3—N4—C22 | 178.3 (2) | C20—C15—C16—C17 | −1.4 (4) |
Co—N3—N4—C22 | −6.0 (3) | O2—C15—C16—C21 | −1.3 (4) |
Co—O1—C1—C6 | 169.84 (16) | C20—C15—C16—C21 | 177.6 (2) |
Co—O1—C1—C2 | −11.6 (3) | C15—C16—C17—C18 | −1.1 (4) |
O1—C1—C2—C3 | −178.2 (2) | C21—C16—C17—C18 | 179.7 (3) |
C6—C1—C2—C3 | 0.3 (3) | C16—C17—C18—C19 | 2.6 (5) |
O1—C1—C2—C7 | 1.0 (4) | C17—C18—C19—C20 | −1.5 (5) |
C6—C1—C2—C7 | 179.5 (2) | C18—C19—C20—C15 | −1.2 (5) |
C7—C2—C3—C4 | −178.5 (2) | O2—C15—C20—C19 | −178.5 (3) |
C1—C2—C3—C4 | 0.7 (4) | C16—C15—C20—C19 | 2.5 (4) |
C2—C3—C4—C5 | −1.2 (4) | N4—N3—C21—C16 | −178.3 (2) |
C3—C4—C5—C6 | 0.6 (4) | Co—N3—C21—C16 | 5.7 (4) |
C4—C5—C6—C1 | 0.4 (4) | C15—C16—C21—N3 | 6.4 (4) |
O1—C1—C6—C5 | 177.8 (2) | C17—C16—C21—N3 | −174.5 (3) |
C2—C1—C6—C5 | −0.8 (4) | N3—N4—C22—C23 | 179.7 (2) |
N2—N1—C7—C2 | −177.2 (2) | N4—C22—C23—C28 | 169.5 (3) |
Co—N1—C7—C2 | 6.5 (3) | N4—C22—C23—C24 | −12.9 (4) |
C3—C2—C7—N1 | −179.5 (2) | C28—C23—C24—C25 | 1.7 (4) |
C1—C2—C7—N1 | 1.3 (4) | C22—C23—C24—C25 | −176.0 (2) |
N1—N2—C8—C9 | 178.1 (2) | C28—C23—C24—Cl3 | −179.22 (19) |
N2—C8—C9—C10 | 17.7 (4) | C22—C23—C24—Cl3 | 3.1 (4) |
N2—C8—C9—C14 | −160.7 (3) | C23—C24—C25—C26 | 0.1 (4) |
C14—C9—C10—C11 | 2.5 (4) | Cl3—C24—C25—C26 | −179.0 (2) |
C8—C9—C10—C11 | −175.9 (3) | C24—C25—C26—C27 | −1.2 (4) |
C14—C9—C10—Cl1 | −176.4 (2) | C25—C26—C27—C28 | 0.2 (4) |
C8—C9—C10—Cl1 | 5.2 (4) | C26—C27—C28—C23 | 1.8 (4) |
C9—C10—C11—C12 | −0.8 (5) | C26—C27—C28—Cl4 | −177.9 (2) |
Cl1—C10—C11—C12 | 178.2 (3) | C24—C23—C28—C27 | −2.7 (4) |
C10—C11—C12—C13 | −1.0 (5) | C22—C23—C28—C27 | 175.1 (2) |
C11—C12—C13—C14 | 0.9 (5) | C24—C23—C28—Cl4 | 176.99 (19) |
C12—C13—C14—C9 | 1.1 (4) | C22—C23—C28—Cl4 | −5.2 (3) |
Hydrogen-bond geometry (Å, º) topCg3 is the centroid of the C1–C6 ring. |
D—H···A | D—H | H···A | D···A | D—H···A |
C27—H27···O1i | 0.93 | 2.35 | 3.114 (3) | 140 |
C25—H25···Cg3ii | 0.93 | 2.86 | 3.647 (3) | 143 |
Symmetry codes: (i) x+1, y, z; (ii) −x+2, −y+1, −z+1. |
Bis(2-{[(2,6-dichlorobenzylidene)hydrazinylidene]methyl}phenolato)copper(II) (II)
top Crystal data top [Cu(C14H9Cl2N2O)2] | Z = 1 |
Mr = 647.80 | F(000) = 327 |
Triclinic, P1 | Dx = 1.626 Mg m−3 |
a = 8.1300 (7) Å | Mo Kα radiation, λ = 0.71073 Å |
b = 8.5072 (11) Å | Cell parameters from 3126 reflections |
c = 9.7386 (13) Å | θ = 4.9–56.5° |
α = 83.240 (4)° | µ = 1.26 mm−1 |
β = 87.646 (3)° | T = 296 K |
γ = 81.533 (4)° | Block, dark-brown |
V = 661.39 (14) Å3 | 0.35 × 0.35 × 0.30 mm |
Data collection top Bruker Kappa APEXII CCD diffractometer | 2708 reflections with I > 2σ(I) |
ω and φ scan | Rint = 0.021 |
Absorption correction: multi-scan (SADABS; Bruker, 2004) | θmax = 28.3°, θmin = 3.3° |
Tmin = 0.637, Tmax = 0.714 | h = −5→10 |
5554 measured reflections | k = −11→11 |
3090 independent reflections | l = −12→12 |
Refinement top Refinement on F2 | Primary atom site location: structure-invariant direct methods |
Least-squares matrix: full | Secondary atom site location: difference Fourier map |
R[F2 > 2σ(F2)] = 0.033 | Hydrogen site location: inferred from neighbouring sites |
wR(F2) = 0.093 | H-atom parameters constrained |
S = 1.05 | w = 1/[σ2(Fo2) + (0.0461P)2 + 0.2934P] where P = (Fo2 + 2Fc2)/3 |
3090 reflections | (Δ/σ)max < 0.001 |
178 parameters | Δρmax = 0.44 e Å−3 |
0 restraints | Δρmin = −0.46 e Å−3 |
Special details top Geometry. All esds (except the esd in the dihedral angle between two l.s. planes) are estimated using the full covariance matrix. The cell esds are taken into account individually in the estimation of esds in distances, angles and torsion angles; correlations between esds in cell parameters are only used when they are defined by crystal symmetry. An approximate (isotropic) treatment of cell esds is used for estimating esds involving l.s. planes. |
Fractional atomic coordinates and isotropic or equivalent isotropic displacement parameters (Å2) top | x | y | z | Uiso*/Ueq | |
Cu | 0.500000 | 0.500000 | 0.500000 | 0.02898 (11) | |
Cl1 | 0.31832 (11) | 0.73971 (12) | 0.01621 (8) | 0.0830 (3) | |
Cl2 | −0.14142 (7) | 0.45455 (7) | 0.33048 (7) | 0.05364 (17) | |
O1 | 0.5954 (2) | 0.30738 (17) | 0.59863 (16) | 0.0435 (4) | |
N1 | 0.4436 (2) | 0.37945 (19) | 0.34549 (16) | 0.0293 (3) | |
N2 | 0.3392 (2) | 0.4457 (2) | 0.23415 (17) | 0.0352 (4) | |
C1 | 0.6593 (3) | 0.1736 (2) | 0.5513 (2) | 0.0338 (4) | |
C2 | 0.6301 (3) | 0.1364 (2) | 0.4182 (2) | 0.0325 (4) | |
C3 | 0.7051 (3) | −0.0117 (3) | 0.3762 (3) | 0.0439 (5) | |
H3 | 0.684470 | −0.036821 | 0.288672 | 0.053* | |
C4 | 0.8071 (3) | −0.1183 (3) | 0.4617 (3) | 0.0519 (6) | |
H4 | 0.857619 | −0.214233 | 0.432071 | 0.062* | |
C5 | 0.8345 (3) | −0.0824 (3) | 0.5923 (3) | 0.0519 (6) | |
H5 | 0.903270 | −0.155502 | 0.650883 | 0.062* | |
C6 | 0.7625 (3) | 0.0588 (3) | 0.6378 (3) | 0.0459 (5) | |
H6 | 0.782038 | 0.079204 | 0.726963 | 0.055* | |
C7 | 0.5198 (3) | 0.2392 (2) | 0.3251 (2) | 0.0321 (4) | |
H7 | 0.500996 | 0.201827 | 0.242030 | 0.038* | |
C8 | 0.2031 (2) | 0.5217 (2) | 0.2710 (2) | 0.0327 (4) | |
H8 | 0.179795 | 0.526076 | 0.364870 | 0.039* | |
C9 | 0.0790 (3) | 0.6043 (2) | 0.1705 (2) | 0.0338 (4) | |
C10 | 0.1164 (3) | 0.7083 (3) | 0.0554 (2) | 0.0456 (5) | |
C11 | −0.0074 (4) | 0.7920 (3) | −0.0292 (3) | 0.0597 (7) | |
H11 | 0.019998 | 0.861210 | −0.105066 | 0.072* | |
C12 | −0.1692 (4) | 0.7730 (3) | −0.0015 (3) | 0.0676 (9) | |
H12 | −0.251592 | 0.830675 | −0.058269 | 0.081* | |
C13 | −0.2130 (3) | 0.6699 (3) | 0.1091 (3) | 0.0589 (7) | |
H13 | −0.323355 | 0.655485 | 0.126954 | 0.071* | |
C14 | −0.0872 (3) | 0.5881 (2) | 0.1931 (2) | 0.0392 (5) | |
Atomic displacement parameters (Å2) top | U11 | U22 | U33 | U12 | U13 | U23 |
Cu | 0.02975 (19) | 0.02729 (17) | 0.02922 (18) | −0.00160 (12) | −0.00773 (12) | −0.00101 (12) |
Cl1 | 0.0648 (5) | 0.1185 (7) | 0.0567 (4) | −0.0217 (5) | 0.0044 (3) | 0.0361 (4) |
Cl2 | 0.0335 (3) | 0.0511 (3) | 0.0743 (4) | −0.0079 (2) | 0.0011 (3) | 0.0025 (3) |
O1 | 0.0645 (11) | 0.0280 (7) | 0.0364 (8) | 0.0034 (7) | −0.0205 (7) | −0.0033 (6) |
N1 | 0.0274 (8) | 0.0338 (8) | 0.0264 (7) | −0.0031 (6) | −0.0058 (6) | −0.0014 (6) |
N2 | 0.0360 (9) | 0.0415 (9) | 0.0273 (8) | −0.0016 (7) | −0.0089 (7) | −0.0033 (7) |
C1 | 0.0346 (11) | 0.0265 (9) | 0.0404 (10) | −0.0063 (8) | −0.0087 (8) | 0.0015 (7) |
C2 | 0.0301 (10) | 0.0297 (9) | 0.0377 (10) | −0.0056 (7) | 0.0007 (8) | −0.0025 (7) |
C3 | 0.0474 (14) | 0.0369 (11) | 0.0465 (12) | −0.0020 (9) | 0.0044 (10) | −0.0079 (9) |
C4 | 0.0484 (14) | 0.0331 (11) | 0.0702 (17) | 0.0059 (10) | 0.0038 (12) | −0.0057 (11) |
C5 | 0.0471 (14) | 0.0335 (11) | 0.0700 (17) | 0.0039 (10) | −0.0135 (12) | 0.0067 (11) |
C6 | 0.0529 (14) | 0.0336 (10) | 0.0498 (13) | −0.0026 (9) | −0.0207 (11) | 0.0037 (9) |
C7 | 0.0328 (10) | 0.0357 (9) | 0.0287 (9) | −0.0066 (8) | 0.0001 (7) | −0.0061 (7) |
C8 | 0.0304 (10) | 0.0414 (10) | 0.0268 (9) | −0.0068 (8) | −0.0060 (7) | −0.0016 (7) |
C9 | 0.0350 (11) | 0.0352 (10) | 0.0317 (10) | −0.0016 (8) | −0.0095 (8) | −0.0068 (8) |
C10 | 0.0534 (15) | 0.0507 (13) | 0.0318 (10) | −0.0051 (11) | −0.0099 (9) | −0.0014 (9) |
C11 | 0.084 (2) | 0.0506 (14) | 0.0422 (13) | −0.0036 (13) | −0.0263 (13) | 0.0042 (11) |
C12 | 0.077 (2) | 0.0517 (15) | 0.0713 (18) | 0.0100 (14) | −0.0474 (16) | −0.0024 (13) |
C13 | 0.0419 (15) | 0.0499 (14) | 0.085 (2) | 0.0029 (11) | −0.0289 (13) | −0.0106 (13) |
C14 | 0.0370 (12) | 0.0330 (10) | 0.0482 (12) | −0.0006 (8) | −0.0126 (9) | −0.0088 (9) |
Geometric parameters (Å, º) top Cu—O1 | 1.8776 (14) | C4—H4 | 0.9300 |
Cu—O1i | 1.8776 (14) | C5—C6 | 1.371 (3) |
Cu—N1 | 2.0211 (16) | C5—H5 | 0.9300 |
Cu—N1i | 2.0211 (16) | C6—H6 | 0.9300 |
Cl1—C10 | 1.722 (3) | C7—H7 | 0.9300 |
Cl2—C14 | 1.737 (2) | C8—C9 | 1.476 (3) |
O1—C1 | 1.306 (2) | C8—H8 | 0.9300 |
N1—C7 | 1.294 (2) | C9—C14 | 1.384 (3) |
N1—N2 | 1.416 (2) | C9—C10 | 1.396 (3) |
N2—C8 | 1.258 (3) | C10—C11 | 1.385 (3) |
C1—C6 | 1.411 (3) | C11—C12 | 1.361 (5) |
C1—C2 | 1.407 (3) | C11—H11 | 0.9300 |
C2—C3 | 1.415 (3) | C12—C13 | 1.376 (5) |
C2—C7 | 1.428 (3) | C12—H12 | 0.9300 |
C3—C4 | 1.365 (3) | C13—C14 | 1.387 (3) |
C3—H3 | 0.9300 | C13—H13 | 0.9300 |
C4—C5 | 1.377 (4) | | |
| | | |
O1—Cu—O1i | 180.00 (10) | C5—C6—H6 | 119.5 |
O1—Cu—N1 | 90.28 (6) | C1—C6—H6 | 119.5 |
O1i—Cu—N1 | 89.72 (6) | N1—C7—C2 | 126.18 (18) |
O1—Cu—N1i | 89.72 (6) | N1—C7—H7 | 116.9 |
O1i—Cu—N1i | 90.28 (6) | C2—C7—H7 | 116.9 |
N1—Cu—N1i | 180.0 | N2—C8—C9 | 122.34 (18) |
C1—O1—Cu | 128.77 (13) | N2—C8—H8 | 118.8 |
C7—N1—N2 | 111.28 (16) | C9—C8—H8 | 118.8 |
C7—N1—Cu | 123.02 (13) | C14—C9—C10 | 116.3 (2) |
N2—N1—Cu | 124.88 (12) | C14—C9—C8 | 119.63 (19) |
C8—N2—N1 | 113.85 (16) | C10—C9—C8 | 124.0 (2) |
O1—C1—C6 | 118.78 (19) | C11—C10—C9 | 121.3 (3) |
O1—C1—C2 | 123.59 (18) | C11—C10—Cl1 | 117.8 (2) |
C6—C1—C2 | 117.63 (19) | C9—C10—Cl1 | 120.87 (18) |
C1—C2—C3 | 119.48 (19) | C12—C11—C10 | 120.1 (3) |
C1—C2—C7 | 122.47 (18) | C12—C11—H11 | 120.0 |
C3—C2—C7 | 117.97 (19) | C10—C11—H11 | 120.0 |
C4—C3—C2 | 121.2 (2) | C11—C12—C13 | 121.1 (2) |
C4—C3—H3 | 119.4 | C11—C12—H12 | 119.4 |
C2—C3—H3 | 119.4 | C13—C12—H12 | 119.4 |
C3—C4—C5 | 119.3 (2) | C14—C13—C12 | 117.9 (3) |
C3—C4—H4 | 120.3 | C14—C13—H13 | 121.1 |
C5—C4—H4 | 120.3 | C12—C13—H13 | 121.1 |
C6—C5—C4 | 121.4 (2) | C13—C14—C9 | 123.3 (2) |
C6—C5—H5 | 119.3 | C13—C14—Cl2 | 118.0 (2) |
C4—C5—H5 | 119.3 | C9—C14—Cl2 | 118.66 (16) |
C5—C6—C1 | 121.0 (2) | | |
| | | |
N1—Cu—O1—C1 | 25.25 (19) | C1—C2—C7—N1 | 4.8 (3) |
N1i—Cu—O1—C1 | −154.75 (19) | C3—C2—C7—N1 | −178.5 (2) |
C7—N1—N2—C8 | 141.33 (19) | N1—N2—C8—C9 | 178.03 (18) |
Cu—N1—N2—C8 | −48.8 (2) | N2—C8—C9—C14 | 134.7 (2) |
Cu—O1—C1—C6 | 163.39 (17) | N2—C8—C9—C10 | −49.5 (3) |
Cu—O1—C1—C2 | −17.0 (3) | C14—C9—C10—C11 | 1.1 (3) |
O1—C1—C2—C3 | 179.9 (2) | C8—C9—C10—C11 | −174.8 (2) |
C6—C1—C2—C3 | −0.5 (3) | C14—C9—C10—Cl1 | 179.69 (17) |
O1—C1—C2—C7 | −3.5 (3) | C8—C9—C10—Cl1 | 3.8 (3) |
C6—C1—C2—C7 | 176.1 (2) | C9—C10—C11—C12 | −0.4 (4) |
C1—C2—C3—C4 | −0.9 (3) | Cl1—C10—C11—C12 | −179.1 (2) |
C7—C2—C3—C4 | −177.7 (2) | C10—C11—C12—C13 | −0.8 (4) |
C2—C3—C4—C5 | 1.4 (4) | C11—C12—C13—C14 | 1.2 (4) |
C3—C4—C5—C6 | −0.6 (4) | C12—C13—C14—C9 | −0.5 (4) |
C4—C5—C6—C1 | −0.9 (4) | C12—C13—C14—Cl2 | −178.6 (2) |
O1—C1—C6—C5 | −179.0 (2) | C10—C9—C14—C13 | −0.6 (3) |
C2—C1—C6—C5 | 1.4 (4) | C8—C9—C14—C13 | 175.5 (2) |
N2—N1—C7—C2 | −177.83 (19) | C10—C9—C14—Cl2 | 177.47 (16) |
Cu—N1—C7—C2 | 12.1 (3) | C8—C9—C14—Cl2 | −6.4 (3) |
Symmetry code: (i) −x+1, −y+1, −z+1. |
Selected geometric parameters (Å, °) for (I) and (II)a topParameter | (I): M = CoII | (II): M = CuII |
M—O1 | 1.8940 (17) | 1.8776 (14) |
M—O2 | 1.8937 (17) | 1.8776 (14)a |
M—N1 | 1.9988 (19) | 2.0211 (16) |
M—N3 | 1.999 (2) | 2.0211 (16)a |
N1—N2 | 1.411 (3) | 1.416 (2) |
N3—N4 | 1.410 (3) | 1.416 (2)a |
C7—N1 | 1.304 (3) | 1.294 (2) |
C8—N2 | 1.251 (3) | 1.258 (3) |
C21—N3 | 1.303 (3) | 1.294 (2)a |
C22—N4 | 1.247 (3) | 1.258 (3)a |
| | |
O1—Co—O2 | 125.33 (8) | 180a |
O1—Co—N1 | 94.06 (7) | 90.28 (6) |
O1—Co—N3 | 112.12 (8) | 89.72 (6) |
O2—Co—N1 | 113.82 (8) | 89.72 (6)a |
O2—Co—N3 | 94.60 (8) | 90.28 (6)a |
N1—Co—N3 | 119.03 (8) | 180a |
Notes: (a) The CuII atom in (II) lies on a centre of inversion so O2 is equivalent to O1, N3 to N1, etc. and are related by the symmetry operation 1 - x, 1 - y, 1 - z. |
Summary of short interatomic contacts (Å) in (I) and (II)a topContact | Distance | Symmetry operation |
(I) | | |
Cl1···Cl3 | 3.4993 (13) | x, -1 + y, z |
Cl3···H7 | 2.70 | x, 1 + y, z |
Cl4···H3 | 2.74 | 2 - x, - y, 1 - z |
C1···H26 | 2.71 | 2 - x, 1 - y, 1 - z |
C6···H26 | 2.76 | 2 - x, 1 - y, 1 - z |
Co···C27 | 3.558 (3) | -1 + x, y, z |
Co···H27 | 3.08 | -1 + x, y, z |
H5···H12 | 2.23 | x, y, -1 + z |
H5···H13 | 2.30 | x, y, -1 + z |
(II) | | |
Cl1···H7 | 2.81 | 1 - x, 1 - y, -z |
C5···C7 | 3.378 (3) | 1 - x, -y, 1 - z |
Cu···Cl2 | 3.2858 (7) | 1 + x, y, z |
Notes: (a) The interatomic distances are calculated in Crystal Explorer 17 (Turner et al., 2017) whereby the X—H bond lengths are adjusted to their neutron values. |
Percentage contributions of interatomic contacts to the Hirshfeld surface for (I) and (II) topContact | | Percentage contribution |
| (I) | (II) |
H···H | 26.2 | 30.5 |
O···H/H···O | 7.9 | 4.2 |
C···H/H···C | 16.7 | 14.5 |
Cl···H/H···Cl | 25.8 | 24.9 |
C···C | 12.0 | 9.8 |
N···H/H··· N | 5.5 | 6.2 |
Cl···Cl | 2.2 | 0.4 |
C···O/O···C | 0.5 | 0.3 |
C···N/N···C | 0.5 | 1.3 |
Cl···O/O···Cl | 0.2 | 0.4 |
C···Cl/Cl···C | 1.9 | 3.9 |
Cl···N/N···Cl | 0.2 | 1.6 |
M···H/H···M | 0.4 | 0.1 |
M···Cl/Cl···M | 0.0 | 1.9 |
Summary of interaction energies (kJ mol-1) calculated for (I) topContact | R (Å) | Eele | Epol | Edis | Erep | Etot |
H27···Coi + | 8.81 | -21.7 | -6.7 | -71.3 | 41.6 | -57.0 |
C27···Coi + | | | | | | |
C27—H27···O1i + | | | | | | |
Cg(C15–C20)···Cg(C23–C28)i | | | | | | |
Cg(C9–C14) ···Cg(C9–C14)ii | 9.60 | -29.6 | -7.6 | -71.9 | 32.5 | -73.5 |
Cg(C1–C6) ···Cg(C1–C6)iii | 10.19 | -23.4 | -5.5 | -58.3 | 29.4 | -56.0 |
Cl4···H3iv + | 10.54 | -12.7 | -1.2 | -26.4 | 19.8 | -21.4 |
Cl1···Cl3iv | | | | | | |
Cl3···H7v | 10.48 | -3.9 | -1.3 | -14.8 | 13.4 | -7.3 |
C1···H26vi + | 9.86 | -37.0 | -8.0 | -84.1 | 48.4 | -79.5 |
C6···H26vi + | | | | | | |
C25–H25···Cg(C1–C6)vi + | | | | | | |
Cg(C23–C28)···Cg(C23–C28)vi | | | | | | |
Symmetry codes: (i) 1 + x, y, z; (ii) 1 - x, - y, 2 - z; (iii) 1 - x, - y, 1 - z; (iv) 2 - x, - y, 1 - z; (v) x, 1 + y, z; (vi) 2 - x, 1 - y, 1 - z. |
Summary of interaction energies (kJ mol-1) calculated for (II) topContact | R (Å) | Eele | Epol | Edis | Erep | Etot |
Cg(C9–C14)···Cg(C9–C14)i | 12.94 | -0.7 | -3.0 | -44.5 | 14.8 | -30.8 |
Cu···Cl2ii | 8.13 | -33.0 | -5.6 | -64.1 | 44.4 | -59.0 |
Cl1···H7iii | 9.74 | -3.7 | -2.5 | -25.7 | 15.4 | -16.0 |
C5···C7iv + | 8.51 | -14.4 | -4.7 | -68.5 | 36.8 | -49.6 |
Cg(C1–C6)···Cg(C1–C6)iv | | | | | | |
Symmetry codes: (i) - x, 1 - y, - z; (ii) 1 + x, y, z; (iii) 1 - x, 1 - y, -z; (iv) 1 - x, - y, 1 - z. |
Acknowledgements
The authors are thankful to the Department of Chemistry, Saurashtra University, Rajkot, Gujarat, India, for access to the chemical synthesis laboratory, to the Sophisticated Analytical Instrumentation Center (SAIC), Tezpur, Asam, India for providing the X-ray intensity data for (I)
and to the Sophisticated Test and Instrumentation Centre (STIC), Kochi, Kerala, India, for the X-ray intensity data for (II)
.
Funding information
Crystallographic research at Sunway University is supported by Sunway University Sdn Bhd (grant No. STR-RCTR-RCCM-001-2019).
References
Altomare, A., Cascarano, G., Giacovazzo, C., Guagliardi, A., Burla, M. C., Polidori, G. & Camalli, M. (1994). J. Appl. Cryst. 27, 435. CrossRef Web of Science IUCr Journals Google Scholar
Annapoorani, S. & Krishnan, C. (2013). J. Chem. Tech. Res. 5, 180–185. CAS Google Scholar
Bagihalli, G. B., Avaji, P. G., Patil, S. A. & Badami, P. S. (2008). Eur. J. Med. Chem. 43, 2639–2649. Web of Science CrossRef PubMed CAS Google Scholar
Brandenburg, K. (2006). DIAMOND. Crystal Impact GbR, Bonn, Germany. Google Scholar
Bruker (2004). APEX2, SAINT and SADABS. Bruker AXS Inc., Madison, Wisconsin, USA. Google Scholar
Chohan, Z. H., Jaffery, M. F. & Supuran, C. T. (2001). Met.-Based Drugs, 8, 95–101. CrossRef CAS Google Scholar
Farrugia, L. J. (2012). J. Appl. Cryst. 45, 849–854. Web of Science CrossRef CAS IUCr Journals Google Scholar
Guo, Z., Xing, R., Liu, S., Zhong, Z., Ji, X., Wang, L. & Li, P. (2007). Carbohydr. Res. 342, 1329–1332. Web of Science CrossRef PubMed CAS Google Scholar
Hsu, C.-Y., Tseng, H.-C., Vandavasi, J. K., Lu, W.-Y., Wang, L.-F., Chiang, M. Y., Lai, Y.-C., Chen, H.-Y. & Chen, H. (2017). RSC Adv. 7, 18851–18860. CSD CrossRef CAS Google Scholar
Liu, E., Zhang, Y. Z., Tan, J., Yang, C., Li, L., Golen, J. A., Rheingold, A. L. & Zhang, G. (2015). Polyhedron, 102, 41–47. CSD CrossRef CAS Google Scholar
Manawar, R. B., Gondaliya, M. B., Mamtora, M. J. & Shah, M. K. (2019). Sci. News 126, 222–247. Google Scholar
Manawar, R. B., Gondaliya, M. B., Shah, M. K., Jotani, M. M. & Tiekink, E. R. T. (2019). Acta Cryst. E75, 1423–1428. CSD CrossRef IUCr Journals Google Scholar
Patti, A., Pedotti, S., Ballistreri, F. P. & Sfrazzetto, G. T. (2009). Molecules, 14, 4312–4325. Web of Science CrossRef PubMed CAS Google Scholar
Pinto, C. B., Dos Santos, L. H. R. & Rodrigues, B. L. (2019). Acta Cryst. C75, 707–716. Web of Science CSD CrossRef IUCr Journals Google Scholar
Prakash, G., Nirmala, M., Ramachandran, R., Viswanathamurthi, P., Malecki, J. G. & Sanmartin, J. (2015). Polyhedron, 89, 62–69. CSD CrossRef CAS Google Scholar
Przybylski, P., Huczynski, A., Pyta, K., Brzezinski, B. & Bartl, F. (2009). Curr. Org. Chem. 13, 124–148. Web of Science CrossRef CAS Google Scholar
Sheldrick, G. M. (2015). Acta Cryst. C71, 3–8. Web of Science CrossRef IUCr Journals Google Scholar
Tan, S. L., Jotani, M. M. & Tiekink, E. R. T. (2019). Acta Cryst. E75, 308–318. Web of Science CrossRef IUCr Journals Google Scholar
Tian, B., He, M., Tan, Z., Tang, S., Hewlett, I., Chen, S., Jin, Y. & Yang, M. (2011). Chem. Biol. Drug Des. 77, 189–198. Web of Science CrossRef CAS PubMed Google Scholar
Tian, B., He, M., Tang, S., Hewlett, I., Tan, Z., Li, J., Jin, Y. & Yang, M. (2009). Bioorg. Med. Chem. Lett. 19, 2162–2167. Web of Science CrossRef PubMed CAS Google Scholar
Turner, M. J., Mckinnon, J. J., Wolff, S. K., Grimwood, D. J., Spackman, P. R., Jayatilaka, D. & Spackman, M. A. (2017). Crystal Explorer 17. The University of Western Australia. Google Scholar
Westrip, S. P. (2010). J. Appl. Cryst. 43, 920–925. Web of Science CrossRef CAS IUCr Journals Google Scholar
Yang, L., Powell, D. R. & Houser, R. P. (2007). Dalton Trans. pp. 955–964. Web of Science CSD CrossRef PubMed CAS Google Scholar
This is an open-access article distributed under the terms of the Creative Commons Attribution (CC-BY) Licence, which permits unrestricted use, distribution, and reproduction in any medium, provided the original authors and source are cited.
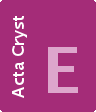 | CRYSTALLOGRAPHIC COMMUNICATIONS |
ISSN: 2056-9890
Open

access