1. Chemical context
The benzoate anion, C7H5O2− is a classic ligand in coordination chemistry, with over 1500 crystal structures reported in the Cambridge Structural Database (version 5.40, updated to February 2020; Groom et al., 2016
) for benzoate complexes of first-row transition metals alone. Functionalized benzoic acid derivatives add further structural variety: for example, –NH2 substituents at the ortho, meta and/or para positions of the benzene ring can form or accept hydrogen bonds with respect to nearby acceptor or donor groups and/or bond as Lewis bases to another metal ion (i.e. as a μ2-N,O or μ3-N,O,O bridging ligand). It should be noted that the presence of amine groups allows for protonation and the possible formation of molecular salts with the aminobenzoic acid acting as the cation.
As part of our ongoing studies in this area (Khosa et al., 2015
), we now describe the syntheses and structures of three molecular salts of protonated 3,4-diaminobenzoic acid, viz. C7H9N2O2·Cl (I)
, C7H9N2O2·Br (II)
and C7H9N2O2·NO3·H2O (III)
. Hirshfeld surface analyses have been performed to gain further insight into the intermolecular interactions.
2. Structural commentary
The contents of the asymmetric units of (I)
(Fig. 1
), (II)
(Fig. 2
) and (III)
(Fig. 3
) confirm them to be molecular salts of 3,4-diaminobenzoic acid (i.e. the C7H9N2O2+ 2-amino-5-carboxyanilinium cation has been formed) and the appropriate strong acid (hydrochloric acid, hydrobromic acid and nitric acid, respectively); compound (III)
also includes a water molecule of crystallization. The neutral organic molecule (C7H8N2O2) is known to crystallize as a zwitterion (Rzaczyńska et al., 2000
) with nominal intramolecular proton transfer from the carboxylic acid to the meta-N atom and presumably exists in the same form in solution, thus the formal acid–base reaction to form the title salts involves proton transfer from the strong acid to the –CO2− carboxylate group of zwitterionic C7H8N2O2 to form a –CO2H carboxylic acid group; atom N1 remains protonated, to result in the C7H9N2O2+ cation.
| Figure 1 The molecular structure of (I) showing 50% displacement ellipsoids. The N—H⋯Cl hydrogen bond is indicated by a double-dashed line. |
| Figure 2 The molecular structure of (II) showing 50% displacement ellipsoids. The N—H⋯Br hydrogen bond is indicated by a double-dashed line. |
| Figure 3 The molecular structure of (III) showing 50% displacement ellipsoids. The O—H⋯O hydrogen bonds are indicated by double-dashed lines. |
The preference for the meta –NH2 group to be protonated in these salts compared to the para –NH2 group can be rationalized in terms of the potential loss of conjugation of the para-N-atom lone pair of electrons with the carboxylic acid grouping via the benzene ring, i.e., a small contribution of a quinoid (C=N+ containing) resonance form to the structure (Lai & Marsh, 1967
): the mean bond lengths for C1—C2, C3—C4, C4—C5 and C1—C6 (single bonds in the quinoid structure) and C2—C3 and C5—C6 (double bonds) are 1.401/1.380, 1.400/1.381 and 1.403/1.373 Å for (I)
, (II)
and (III)
, respectively (global averages = 1.401/1.378 Å). These data compare very well to the equivalent values of 1.399/1.375 Å established over 50 years ago from Weissenberg data for p-aminobenzoic acid (Lai & Marsh, 1967
).
This electronic effect is also no doubt reflected in the fact that the C4—N2 (para) bond in the title compounds is notably shorter than the C3—N1 (meta) bond [distances in (I)
= 1.378 (2) and 1.4640 (19), respectively; (II)
= 1.387 (6) and 1.468 (6); (III)
= 1.386 (5) and 1.457 (5) Å]. Even so, it may be noted that the bond-angle sums about N2 are 348.0, 340.4, and 339.2° for (I)
, (II)
and (III)
, respectively, suggesting a tendency towards sp3 hybridization (and presumably lone-pair localization) for the nitrogen atom in each case: it also correlates with the fact that N2 accepts a hydrogen bond in the crystal of (III)
(vide infra).
For each structure, the carboxylic acid group shows the expected clear distinction between the C7—O1H [(I) = 1.296 (2), (II)
= 1.326 (5), (III)
= 1.323 (6)Å] and C7=O2 [(I) = 1.252 (2), (II)
= 1.216 (5), (III)
= 1.232 (5) Å] bond lengths. The degree of twist of the –CO2H group with respect to the benzene ring is similar in the three salts [the angles between the mean planes passing through atoms C1–C6 and O1/O2/C7 are (I)
= 6.39 (16), (II)
= 0.5 (4), (III)
= 3.8 (5)°].
3. Supramolecular features
In the crystal of (I)
, the cations are connected into carboxylic acid inversion dimers via pairwise O—H⋯O hydrogen bonds (Table 1
), thereby generating classical R22(8) loops. All five N—H groups link to a nearby chloride ion: the H⋯Cl contacts from the protonated –N1H3+ moiety (mean = 2.35 Å) are substantially shorter than those arising from the unprotonated –N2H2 group (mean = 2.70 Å). As a result, the chloride ion accepts five N—H⋯Cl bonds from four cations (one cation bonds from both N1 and N2) in an irregular geometry (Fig. 4
). The overall packing for (I)
results in corrugated (100) sheets of chloride ions bridged by the carboxylic acid dimers into a three-dimensional supramolecular network (Fig. 5
).
D—H⋯A | D—H | H⋯A | D⋯A | D—H⋯A | O1—H1O⋯O2i | 0.74 (3) | 1.88 (3) | 2.6169 (18) | 170 (3) | N1—H1N⋯Cl1ii | 0.90 (2) | 2.32 (2) | 3.1578 (14) | 153.4 (17) | N1—H2N⋯Cl1 | 0.91 (2) | 2.25 (2) | 3.1576 (15) | 172.1 (18) | N1—H3N⋯Cl1iii | 0.90 (2) | 2.49 (2) | 3.1722 (14) | 132.9 (16) | N2—H4N⋯Cl1iv | 0.86 (2) | 2.72 (2) | 3.2382 (15) | 120.3 (17) | N2—H5N⋯Cl1iii | 0.81 (2) | 2.68 (2) | 3.4852 (15) | 176 (2) | Symmetry codes: (i) -x+1, -y+2, -z+1; (ii) ; (iii) ; (iv) . | |
| Figure 4 Environment of the chloride ion in the structure of (I) with N—H⋯Cl hydrogen bonds indicated by double-dashed lines. Symmetry codes: (i) − x, y − , − z; (ii) x, 1 − y, z − ; (iii) − x, + y, − z. |
| Figure 5 The packing for (I) viewed down [001]. The hydrogen bonds linking the carboxylic-acid inversion dimers are shown as double-dashed lines. |
Rather than carboxylic acid inversion dimers, the packing for (II)
features O—H⋯Br hydrogen bonds as well as N—H⋯Br and N—H⋯O contacts (Table 2
). The bromide ion (Fig. 6
) is five-coordinated in an irregular geometry by four N—H⋯Br and one O—H⋯Br link arising from five different cations. The N1—H2N⋯O2 interaction from the protonated –NH3+ group to the C=O bond of the carboxylic acid generates [010] C(7) chains of cations, with adjacent ions in the chain related by the 21 screw axis. When all the hydrogen bonds are considered together, the packing for (II)
can be described as a three-dimensional supramolecular network of undulating (100) sheets of bromide ions alternating with the organic cations (Fig. 7
). A notably short C—H⋯O interaction (H⋯O = 2.23 Å), which reinforces the C(7) chain of cations, is also observed.
D—H⋯A | D—H | H⋯A | D⋯A | D—H⋯A | O1—H1O⋯Br1i | 0.79 (7) | 2.42 (7) | 3.199 (3) | 169 (6) | N1—H1N⋯Br1 | 0.73 (6) | 2.70 (7) | 3.404 (5) | 163 (6) | N1—H2N⋯O2ii | 0.90 (6) | 1.90 (6) | 2.787 (5) | 168 (5) | N1—H3N⋯Br1iii | 0.85 (6) | 2.49 (6) | 3.333 (5) | 171 (5) | N2—H4N⋯Br1iv | 0.81 (7) | 2.98 (7) | 3.705 (5) | 150 (5) | N2—H5N⋯Br1v | 0.82 (6) | 2.98 (6) | 3.513 (4) | 125 (5) | C2—H2⋯O2ii | 0.95 | 2.23 | 3.024 (5) | 140 | Symmetry codes: (i) -x+1, -y+1, -z; (ii) ; (iii) x, y, z+1; (iv) ; (v) -x+2, -y+1, -z+1. | |
| Figure 6 Environment of the bromide ion in the structure of (II) with N—H⋯Br and O—H⋯Br hydrogen bonds indicated by double-dashed lines. Symmetry codes: (i) x, y, z − 1; (ii) 2 − x, 1 − y, 1 − z; (iii) 2 − x, + y, − z; (iv) 1 − x, 1 − y, −z. |
| Figure 7 The packing for (II) viewed down [001]. |
The directional intermolecular interactions in (III)
(Table 3
) include Oc—H⋯On, Ow—H⋯(On,On), N—H⋯Oc, N—H⋯N and N—H⋯On (c = carboxylic acid, n = nitrate, w = water) hydrogen bonds. The O atoms of the nitrate ion collectively accept four simple and one bifurcated hydrogen bond (Fig. 8
) from three cations and two water molecules. As in (II)
, an N1—H2N⋯O2 hydrogen bond in (III)
generates C(7) chains of cations propagating in [010] with adjacent ions related by the screw axis but an N1—H3N⋯N2 interaction also occurs; by itself it leads to [100] C(5) chains with adjacent ions related by translation; together, (001) hydrogen-bonded sheets of cations arise. When the nitrate ion and water molecules are taken together, undulating hydrogen-bonded chains propagating in the [010] direction arise. Collectively, the packing in (III)
(Fig. 9
) can be described as alternating (001) slabs of nitrate anions + water molecules and organic cations arising from a three-dimensional supramolecular network of hydrogen bonds.
D—H⋯A | D—H | H⋯A | D⋯A | D—H⋯A | N1—H1N⋯O6i | 0.91 | 1.87 | 2.764 (5) | 165 | N1—H2N⋯O2ii | 0.91 | 1.91 | 2.808 (5) | 168 | N1—H3N⋯N2iii | 0.91 | 2.32 | 3.094 (6) | 143 | N1—H3N⋯O6iv | 0.91 | 2.33 | 2.862 (5) | 118 | N2—H4N⋯O4i | 0.91 (6) | 2.07 (6) | 2.972 (5) | 169 (5) | N2—H5N⋯O5v | 0.83 (6) | 2.34 (7) | 3.129 (5) | 159 (6) | O1—H1O⋯O3 | 0.88 (6) | 1.79 (6) | 2.662 (5) | 170 (6) | O6—H2O⋯O5 | 0.86 | 2.02 | 2.884 (5) | 176 | O6—H3O⋯O3vi | 0.96 | 2.26 | 2.920 (5) | 125 | O6—H3O⋯O4vi | 0.96 | 2.33 | 3.068 (5) | 133 | C2—H2⋯O2ii | 0.95 | 2.54 | 3.285 (5) | 135 | Symmetry codes: (i) x-1, y, z-1; (ii) ; (iii) x+1, y, z; (iv) x, y, z-1; (v) ; (vi) . | |
| Figure 8 Environment of the nitrate ion in the structure of (III) with N—H⋯O and O—H⋯O hydrogen bonds indicated by double-dashed lines. Symmetry codes: (i) 1 + x, y, 1 + z; (ii) 2 − x, + y, 2 − z; (iii) −x, y − ; 1 − z. |
| Figure 9 The packing for (III) viewed down [100]. |
The three structures feature weak aromatic π–π stacking (Table 4
). In each case, infinite stacks of molecules, with considerable slippage between adjacent benzene rings, arise: these stacks propagate in the [001], [001] and [100] directions for (I)
, (II)
and (III)
, respectively. A crystallographic c-glide generates the stacks in (I)
and (II)
, whereas in (III)
adjacent molecules are related by simple translation.
Compound | Cg⋯Cg (Å) | α (°) | slippage (Å) | symmetry | (I) | 3.8895 (9) | 1.79 (7) | 1.902 | x, 1 − y, z − ![[{1\over 2}]](teximages/xi2026fi17.gif) | (I) | 3.8895 (9) | 1.79 (7) | 1.822 | x, 1 − y, z + ![[{1\over 2}]](teximages/xi2026fi17.gif) | (II) | 3.736 (3) | 1.5 (2) | 2.035 | x, − y, z − ![[{1\over 2}]](teximages/xi2026fi17.gif) | (II) | 3.736 (3) | 1.5 (2) | 1.954 | x, − y, z + ![[{1\over 2}]](teximages/xi2026fi17.gif) | (III) | 3.890 (2) | 0.0 (2) | 2.105 | x − 1, y, z | (III) | 3.890 (2) | 0.0 (2) | 2.105 | x + 1, y, z | | |
4. Hirshfeld surface analyses
In order to gain further insight into the intermolecular interactions in (I)
, (II)
and (III)
, their Hirshfeld surfaces and two-dimensional fingerprint plots were calculated using CrystalExplorer (Turner et al., 2017
) following the approach recently described by Tan et al. (2019
). The Hirshfeld surfaces of the cations in (I)
, (II)
and (III)
(see supplementary materials) show the expected red spots of varying intensity corresponding to close contacts resulting from the hydrogen bonds described above. The percentage contributions of the different type of contacts to the surfaces (Table 5
) call for some comment. Despite their different packing motifs, especially the presence of pairwise O—H⋯O hydrogen bonds in (I)
and O—H⋯Br and N—H⋯O interactions in (II)
, the contact percentages for the C7H9N2O2+ cations in (I)
and (II)
are strikingly similar, being mostly within 1% of each other. It may be seen that H⋯H (van der Waals) contacts dominate, followed by H⋯X (X = Cl, Br), H⋯O (donor) and then O⋯H (acceptor), with other contacts playing a minor role. The contact percentage data for (III)
are decidedly different with H⋯O (donor) (32.2%) dominating and H⋯H (23.3%) relegated to second place, followed by O⋯H (acceptor) (12.6%). Despite the N—H⋯N hydrogen bond in (III)
, the H⋯N contact percentages barely differ for the three compounds. The contact percentages for the anions in (I)
and (II)
(Table 5
) show them to be essentially `saturated' by their hydrogen bonds, despite the irregular coordination geometries.
Interaction | (I) | (II) | (III) | H⋯H | 30.3 | 30.7 | 23.3 | H⋯Xa | 14.5 | 15.2 | – | H⋯O (donor) | 10.6 | 10.0 | 32.2 | H⋯C | 6.8 | 6.7 | 5.8 | H⋯N | 1.9 | 2.2 | 2.3 | C⋯C | 6.7 | 6.8 | 6.4 | C⋯H | 8.6 | 8.6 | 9.0 | C⋯O | 2.2 | 2.4 | 2.9 | N⋯H | 2.1 | 2.7 | 2.1 | O⋯H (acceptor) | 13.3 | 11.6 | 12.6 | Xa⋯H | 99.8 | 98.7 | – | Note: (a) For (I) , X = Cl; for (II) X = Br. | |
The fingerprint plot for the cation in (I)
(Fig. 10
) of outward (i.e. non-reciprocal) contacts shows three prominent features: the spike ending at (di, de) = (∼0.76, ∼1.36 Å) and extending backwards corresponds to the short intermolecular H⋯Cl contacts associated with the N—H⋯Cl hydrogen bonds. The pronounced (0.65, 1.00 Å) feature equates with the H⋯O (donor) contact of the O—H⋯O hydrogen bond and that at (1.00, 0.65 Å) is associated with the H⋯O (acceptor) contact. The fingerprint plot for the cation in (II)
(Fig. 11
) shows the equivalent three spikes ending at (0.76, 1.45), (0.72, 1.08) and (1.06, 0.72 Å): the greater value of de for the first of these presumably reflects the larger size of the bromide ion in (II)
compared to the chloride ion in (I)
. The fingerprint plot for the cation in (III)
(Fig. 12
) naturally lacks the H⋯X (X = Cl, Br) features and has a more symmetric appearance, with the spike at (0.68, 1.02) equating to H⋯O (donor) and that at (1.08, 0.74 Å) equating to the O⋯H (acceptor) contact. The H⋯N (donor) contact is just perceptible as a shoulder-like feature terminating at (0.94, 1.30 Å) but mostly superimposed on the tail of the H⋯O spike. The `wing' like fingerprint plot for the chloride ion in (I)
(Fig. 13
) looks radically different to that of the cation (Fig. 10
) although the end-point at (1.38, 0.77 Å) of the sweeping feature corresponds well with the H⋯Cl contact for the cation. The fingerprint plot for the bromide ion in (II)
(Fig. 14
) with its sweeping feature terminating at (1.45, 0.77 Å) shows similar correspondence with the H⋯Br spike for the cation in (II)
(Fig. 11
).
| Figure 10 Hirshfeld fingerprint plot for the C7H9N2O2+ cation in (I) . |
| Figure 11 Hirshfeld fingerprint plot for the C7H9N2O2+ cation in (II) . |
| Figure 12 Hirshfeld fingerprint plot for the C7H9N2O2+ cation in (III) . |
| Figure 13 Hirshfeld fingerprint plot for the chloride anion in (I) . |
| Figure 14 Hirshfeld fingerprint plot for the bromide anion in (II) . |
5. Database survey
So far as we are aware, no molecular salts containing the C7H9N2O2+ cation have been structurally characterized up to this point. A search of the Cambridge Structural Database (Version 5.40, updated to February 2020; Groom et al., 2016
) yielded five complexes of the 3,4-diaminobenzoate anion (i.e. C7H7N2O2−) with sodium (CCDC refcode BEHJEE; Rzaczyńska et al., 2003
), zinc (MIWSES; Fernández-Palacio et al., 2014
), tin (XUPRUV and XUPSAC; Pruchnik et al., 2002
) and neodymium (YENKOR; Rzaczyńska et al., 1994
). As noted above, the structure of the zwitterionic free molecule of C7H8N2O2 is known (VODWIU; Rzaczyńska et al., 2000
). Interestingly, the neutral, non-zwitterionic form of C7H8N2O2 has been co-crystallized with an organo-rhenium compound and other species (DONDUH; Davies et al., 2014
). In VODWIU, the –CO2− group accepts several N—H⋯O hydrogen bonds while in DONDUH pairwise carboxylic-acid inversion dimers are formed. The different possible structures of the organic species are shown in Fig. 15
.
| Figure 15 Different structures based on 3,4-diaminobenzoic acid: (a) neutral C7H8N2O2 molecule as found in DONDUH; (b) zwitterion in VODWIU; (c) quinoid resonance form of the C7H9N2O2+ cation in the title compounds (see text and compare scheme 1); (d) C7H7N2O2− anion as found in the metal complexes noted in the text. |
7. Refinement
Crystal data, data collection and structure refinement details are summarized in Table 6
. For each structure, the N-bound H atoms were located in difference maps and their positions were freely refined. The C-bound H atoms were geometrically placed (C—H = 0.95 Å) and refined as riding atoms. The water H atoms in (III)
were located in difference maps and refined as riding atoms in their as-found relative locations. The constraint Uiso(H) = 1.2Ueq(carrier) was applied in all cases. The crystal used for the data collection of (II)
was twinned by rotation about [100] in reciprocal space in a 0.4896 (15):0.5104 (15) ratio and data merging was not performed (i.e. HKLF 5 refinement). The absolute structure of (III)
could not be determined based on the refinement reported here.
| (I) | (II) | (III) | Crystal data | Chemical formula | C7H9N2O2+·Cl− | C7H9N2O2+·Br− | C7H9N2O2+·NO3−·H2O | Mr | 188.61 | 233.07 | 233.19 | Crystal system, space group | Monoclinic, C2/c | Monoclinic, P21/c | Monoclinic, P21 | Temperature (K) | 100 | 100 | 100 | a, b, c (Å) | 28.0521 (6), 8.0469 (2), 7.2511 (2) | 12.1190 (7), 11.0708 (5), 6.3795 (3) | 3.8899 (3), 9.8238 (6), 12.5799 (9) | β (°) | 91.309 (2) | 103.047 (6) | 98.673 (7) | V (Å3) | 1636.38 (7) | 833.82 (8) | 475.22 (6) | Z | 8 | 4 | 2 | Radiation type | Mo Kα | Mo Kα | Mo Kα | μ (mm−1) | 0.43 | 4.89 | 0.14 | Crystal size (mm) | 0.41 × 0.26 × 0.04 | 0.25 × 0.12 × 0.04 | 0.20 × 0.10 × 0.05 | | Data collection | Diffractometer | Rigaku Mercury CCD | Rigaku Mercury CCD | Rigaku Mercury CCD | Absorption correction | Gaussian (CrysAlis PRO; Rigaku, 2017 ) | Multi-scan (CrysAlis PRO; Rigaku, 2017 ) | Multi-scan (CrysAlis PRO; Rigaku, 2017 ) | Tmin, Tmax | 0.624, 1.000 | 0.538, 1.000 | 0.861, 1.000 | No. of measured, independent and observed [I > 2σ(I)] reflections | 13456, 1865, 1765 | 3343, 3343, 3031 | 10770, 2157, 2100 | Rint | 0.035 | ? | 0.067 | (sin θ/λ)max (Å−1) | 0.649 | 0.649 | 0.649 | | Refinement | R[F2 > 2σ(F2)], wR(F2), S | 0.031, 0.093, 1.13 | 0.036, 0.100, 1.14 | 0.058, 0.149, 1.15 | No. of reflections | 1865 | 3343 | 2157 | No. of parameters | 127 | 128 | 155 | No. of restraints | 0 | 0 | 1 | H-atom treatment | H atoms treated by a mixture of independent and constrained refinement | H atoms treated by a mixture of independent and constrained refinement | H atoms treated by a mixture of independent and constrained refinement | Δρmax, Δρmin (e Å−3) | 0.45, −0.27 | 1.01, −0.70 | 0.48, −0.37 | Computer programs: CrysAlis PRO (Rigaku, 2017 ), SHELXS97 (Sheldrick, 2008 ), SHELXL2014/7 (Sheldrick, 2015 ), ORTEP-3 for Windows (Farrugia, 2012 ) and publCIF (Westrip, 2010 ). | |
Supporting information
For all structures, data collection: CrysAlis PRO (Rigaku, 2017); cell refinement: CrysAlis PRO (Rigaku, 2017); data reduction: CrysAlis PRO (Rigaku, 2017); program(s) used to solve structure: SHELXS97 (Sheldrick, 2008); program(s) used to refine structure: SHELXL2014/7 (Sheldrick, 2015); molecular graphics: ORTEP-3 for Windows (Farrugia, 2012); software used to prepare material for publication: publCIF (Westrip, 2010).
2-Amino-5-carboxyanilinium chloride (I)
top Crystal data top C7H9N2O2+·Cl− | F(000) = 784 |
Mr = 188.61 | Dx = 1.531 Mg m−3 |
Monoclinic, C2/c | Mo Kα radiation, λ = 0.71073 Å |
a = 28.0521 (6) Å | Cell parameters from 8736 reflections |
b = 8.0469 (2) Å | θ = 2.9–30.1° |
c = 7.2511 (2) Å | µ = 0.43 mm−1 |
β = 91.309 (2)° | T = 100 K |
V = 1636.38 (7) Å3 | Plate, brown |
Z = 8 | 0.41 × 0.26 × 0.04 mm |
Data collection top Rigaku Mercury CCD diffractometer | 1765 reflections with I > 2σ(I) |
ω scans | Rint = 0.035 |
Absorption correction: gaussian (CrysAlisPRO; Rigaku, 2017) | θmax = 27.5°, θmin = 2.6° |
Tmin = 0.624, Tmax = 1.000 | h = −36→36 |
13456 measured reflections | k = −10→10 |
1865 independent reflections | l = −9→9 |
Refinement top Refinement on F2 | Primary atom site location: structure-invariant direct methods |
Least-squares matrix: full | Hydrogen site location: mixed |
R[F2 > 2σ(F2)] = 0.031 | H atoms treated by a mixture of independent and constrained refinement |
wR(F2) = 0.093 | w = 1/[σ2(Fo2) + (0.0434P)2 + 2.8134P] where P = (Fo2 + 2Fc2)/3 |
S = 1.13 | (Δ/σ)max = 0.002 |
1865 reflections | Δρmax = 0.45 e Å−3 |
127 parameters | Δρmin = −0.27 e Å−3 |
0 restraints | |
Special details top Geometry. All esds (except the esd in the dihedral angle between two l.s. planes) are estimated using the full covariance matrix. The cell esds are taken into account individually in the estimation of esds in distances, angles and torsion angles; correlations between esds in cell parameters are only used when they are defined by crystal symmetry. An approximate (isotropic) treatment of cell esds is used for estimating esds involving l.s. planes. |
Fractional atomic coordinates and isotropic or equivalent isotropic displacement parameters (Å2) top | x | y | z | Uiso*/Ueq | |
C1 | 0.42198 (5) | 0.7087 (2) | 0.6424 (2) | 0.0132 (3) | |
C2 | 0.37721 (5) | 0.7561 (2) | 0.7037 (2) | 0.0124 (3) | |
H2 | 0.3696 | 0.8704 | 0.7173 | 0.015* | |
C3 | 0.34405 (5) | 0.6353 (2) | 0.7443 (2) | 0.0115 (3) | |
C4 | 0.35376 (5) | 0.46541 (19) | 0.7258 (2) | 0.0118 (3) | |
C5 | 0.39962 (5) | 0.4200 (2) | 0.6688 (2) | 0.0132 (3) | |
H5 | 0.4077 | 0.3058 | 0.6586 | 0.016* | |
C6 | 0.43292 (6) | 0.5395 (2) | 0.6276 (2) | 0.0139 (3) | |
H6 | 0.4636 | 0.5067 | 0.5887 | 0.017* | |
C7 | 0.45674 (6) | 0.8354 (2) | 0.5858 (2) | 0.0150 (3) | |
N1 | 0.29675 (5) | 0.68573 (18) | 0.8047 (2) | 0.0126 (3) | |
H1N | 0.2945 (7) | 0.797 (3) | 0.818 (3) | 0.015* | |
H2N | 0.2748 (7) | 0.653 (3) | 0.717 (3) | 0.015* | |
H3N | 0.2895 (7) | 0.641 (3) | 0.914 (3) | 0.015* | |
N2 | 0.31909 (5) | 0.34702 (18) | 0.7525 (2) | 0.0158 (3) | |
H4N | 0.3282 (7) | 0.246 (3) | 0.768 (3) | 0.019* | |
H5N | 0.2970 (8) | 0.371 (3) | 0.817 (3) | 0.019* | |
O1 | 0.44480 (5) | 0.98914 (17) | 0.6117 (2) | 0.0226 (3) | |
H1O | 0.4634 (9) | 1.042 (3) | 0.570 (3) | 0.027* | |
O2 | 0.49517 (4) | 0.79238 (15) | 0.51547 (18) | 0.0203 (3) | |
Cl1 | 0.22232 (2) | 0.53660 (4) | 0.51641 (5) | 0.01266 (14) | |
Atomic displacement parameters (Å2) top | U11 | U22 | U33 | U12 | U13 | U23 |
C1 | 0.0110 (7) | 0.0134 (8) | 0.0153 (7) | −0.0017 (6) | 0.0015 (5) | 0.0010 (6) |
C2 | 0.0130 (7) | 0.0109 (7) | 0.0132 (7) | −0.0002 (6) | 0.0004 (5) | 0.0004 (6) |
C3 | 0.0107 (7) | 0.0131 (7) | 0.0108 (7) | 0.0007 (6) | 0.0011 (5) | −0.0002 (6) |
C4 | 0.0128 (7) | 0.0123 (8) | 0.0104 (7) | −0.0010 (5) | −0.0002 (5) | 0.0013 (5) |
C5 | 0.0144 (7) | 0.0107 (7) | 0.0145 (7) | 0.0019 (6) | 0.0011 (5) | 0.0001 (6) |
C6 | 0.0111 (7) | 0.0150 (8) | 0.0157 (8) | 0.0012 (6) | 0.0019 (6) | 0.0003 (6) |
C7 | 0.0129 (7) | 0.0141 (8) | 0.0180 (8) | −0.0013 (6) | 0.0015 (6) | 0.0014 (6) |
N1 | 0.0124 (6) | 0.0106 (7) | 0.0149 (7) | 0.0001 (5) | 0.0032 (5) | 0.0008 (5) |
N2 | 0.0144 (7) | 0.0094 (7) | 0.0236 (7) | 0.0003 (5) | 0.0050 (5) | 0.0014 (6) |
O1 | 0.0181 (6) | 0.0132 (6) | 0.0370 (8) | −0.0040 (5) | 0.0096 (5) | 0.0020 (5) |
O2 | 0.0130 (5) | 0.0189 (6) | 0.0294 (7) | −0.0015 (5) | 0.0074 (5) | 0.0026 (5) |
Cl1 | 0.0148 (2) | 0.0094 (2) | 0.0138 (2) | 0.00125 (12) | 0.00239 (14) | 0.00038 (12) |
Geometric parameters (Å, º) top C1—C2 | 1.395 (2) | C5—H5 | 0.9500 |
C1—C6 | 1.401 (2) | C6—H6 | 0.9500 |
C1—C7 | 1.475 (2) | C7—O2 | 1.252 (2) |
C2—C3 | 1.382 (2) | C7—O1 | 1.296 (2) |
C2—H2 | 0.9500 | N1—H1N | 0.90 (2) |
C3—C4 | 1.401 (2) | N1—H2N | 0.91 (2) |
C3—N1 | 1.4640 (19) | N1—H3N | 0.90 (2) |
C4—N2 | 1.378 (2) | N2—H4N | 0.86 (2) |
C4—C5 | 1.408 (2) | N2—H5N | 0.81 (2) |
C5—C6 | 1.378 (2) | O1—H1O | 0.74 (3) |
| | | |
C2—C1—C6 | 119.38 (14) | C5—C6—H6 | 119.6 |
C2—C1—C7 | 120.35 (15) | C1—C6—H6 | 119.6 |
C6—C1—C7 | 120.22 (14) | O2—C7—O1 | 123.41 (15) |
C3—C2—C1 | 119.42 (15) | O2—C7—C1 | 120.19 (15) |
C3—C2—H2 | 120.3 | O1—C7—C1 | 116.40 (14) |
C1—C2—H2 | 120.3 | C3—N1—H1N | 111.9 (13) |
C2—C3—C4 | 122.18 (14) | C3—N1—H2N | 108.2 (12) |
C2—C3—N1 | 119.22 (14) | H1N—N1—H2N | 108.3 (18) |
C4—C3—N1 | 118.59 (14) | C3—N1—H3N | 112.1 (13) |
N2—C4—C3 | 121.45 (14) | H1N—N1—H3N | 106.6 (19) |
N2—C4—C5 | 120.93 (15) | H2N—N1—H3N | 109.6 (18) |
C3—C4—C5 | 117.53 (14) | C4—N2—H4N | 117.6 (14) |
C6—C5—C4 | 120.72 (15) | C4—N2—H5N | 118.0 (16) |
C6—C5—H5 | 119.6 | H4N—N2—H5N | 112 (2) |
C4—C5—H5 | 119.6 | C7—O1—H1O | 107.7 (19) |
C5—C6—C1 | 120.73 (14) | | |
| | | |
C6—C1—C2—C3 | −1.6 (2) | C3—C4—C5—C6 | −2.0 (2) |
C7—C1—C2—C3 | 175.73 (14) | C4—C5—C6—C1 | 0.3 (2) |
C1—C2—C3—C4 | −0.1 (2) | C2—C1—C6—C5 | 1.5 (2) |
C1—C2—C3—N1 | −178.67 (14) | C7—C1—C6—C5 | −175.82 (15) |
C2—C3—C4—N2 | −174.64 (15) | C2—C1—C7—O2 | −173.60 (15) |
N1—C3—C4—N2 | 3.9 (2) | C6—C1—C7—O2 | 3.7 (2) |
C2—C3—C4—C5 | 1.9 (2) | C2—C1—C7—O1 | 5.8 (2) |
N1—C3—C4—C5 | −179.57 (13) | C6—C1—C7—O1 | −176.96 (16) |
N2—C4—C5—C6 | 174.58 (15) | | |
Hydrogen-bond geometry (Å, º) top D—H···A | D—H | H···A | D···A | D—H···A |
O1—H1O···O2i | 0.74 (3) | 1.88 (3) | 2.6169 (18) | 170 (3) |
N1—H1N···Cl1ii | 0.90 (2) | 2.32 (2) | 3.1578 (14) | 153.4 (17) |
N1—H2N···Cl1 | 0.91 (2) | 2.25 (2) | 3.1576 (15) | 172.1 (18) |
N1—H3N···Cl1iii | 0.90 (2) | 2.49 (2) | 3.1722 (14) | 132.9 (16) |
N2—H4N···Cl1iv | 0.86 (2) | 2.72 (2) | 3.2382 (15) | 120.3 (17) |
N2—H5N···Cl1iii | 0.81 (2) | 2.68 (2) | 3.4852 (15) | 176 (2) |
Symmetry codes: (i) −x+1, −y+2, −z+1; (ii) −x+1/2, y+1/2, −z+3/2; (iii) x, −y+1, z+1/2; (iv) −x+1/2, y−1/2, −z+3/2. |
2-Amino-5-carboxyanilinium bromide (II)
top Crystal data top C7H9N2O2+·Br− | F(000) = 464 |
Mr = 233.07 | Dx = 1.857 Mg m−3 |
Monoclinic, P21/c | Mo Kα radiation, λ = 0.71073 Å |
a = 12.1190 (7) Å | Cell parameters from 8521 reflections |
b = 11.0708 (5) Å | θ = 3.4–30.1° |
c = 6.3795 (3) Å | µ = 4.89 mm−1 |
β = 103.047 (6)° | T = 100 K |
V = 833.82 (8) Å3 | Lath, pale brown |
Z = 4 | 0.25 × 0.12 × 0.04 mm |
Data collection top Rigaku Mercury CCD diffractometer | 3031 reflections with I > 2σ(I) |
ω scans | θmax = 27.5°, θmin = 2.5° |
Absorption correction: multi-scan (CrysAlisPRO; Rigaku, 2017) | h = −15→15 |
Tmin = 0.538, Tmax = 1.000 | k = −14→14 |
3343 measured reflections | l = −8→8 |
3343 independent reflections | |
Refinement top Refinement on F2 | Primary atom site location: structure-invariant direct methods |
Least-squares matrix: full | Hydrogen site location: mixed |
R[F2 > 2σ(F2)] = 0.036 | H atoms treated by a mixture of independent and constrained refinement |
wR(F2) = 0.100 | w = 1/[σ2(Fo2) + (0.0423P)2 + 2.9863P] where P = (Fo2 + 2Fc2)/3 |
S = 1.14 | (Δ/σ)max = 0.001 |
3343 reflections | Δρmax = 1.01 e Å−3 |
128 parameters | Δρmin = −0.69 e Å−3 |
0 restraints | |
Special details top Geometry. All esds (except the esd in the dihedral angle between two l.s. planes) are estimated using the full covariance matrix. The cell esds are taken into account individually in the estimation of esds in distances, angles and torsion angles; correlations between esds in cell parameters are only used when they are defined by crystal symmetry. An approximate (isotropic) treatment of cell esds is used for estimating esds involving l.s. planes. |
Refinement. Refined as a two-component twin |
Fractional atomic coordinates and isotropic or equivalent isotropic displacement parameters (Å2) top | x | y | z | Uiso*/Ueq | |
C1 | 0.5791 (4) | 0.3232 (4) | 0.3020 (7) | 0.0114 (9) | |
C2 | 0.6253 (4) | 0.4394 (4) | 0.3348 (7) | 0.0105 (8) | |
H2 | 0.5781 | 0.5085 | 0.3035 | 0.013* | |
C3 | 0.7405 (4) | 0.4520 (4) | 0.4131 (7) | 0.0093 (8) | |
C4 | 0.8130 (4) | 0.3529 (4) | 0.4643 (7) | 0.0110 (9) | |
C5 | 0.7646 (4) | 0.2369 (4) | 0.4251 (7) | 0.0113 (9) | |
H5 | 0.8119 | 0.1677 | 0.4535 | 0.014* | |
C6 | 0.6494 (4) | 0.2226 (4) | 0.3460 (7) | 0.0122 (9) | |
H6 | 0.6181 | 0.1438 | 0.3216 | 0.015* | |
C7 | 0.4558 (4) | 0.3082 (4) | 0.2165 (7) | 0.0107 (9) | |
N1 | 0.7876 (4) | 0.5748 (3) | 0.4373 (8) | 0.0110 (8) | |
H1N | 0.816 (5) | 0.593 (5) | 0.352 (10) | 0.013* | |
H2N | 0.729 (5) | 0.627 (5) | 0.409 (9) | 0.013* | |
H3N | 0.815 (5) | 0.590 (5) | 0.569 (10) | 0.013* | |
N2 | 0.9285 (4) | 0.3686 (4) | 0.5425 (7) | 0.0155 (9) | |
H4N | 0.959 (5) | 0.304 (6) | 0.578 (9) | 0.019* | |
H5N | 0.951 (5) | 0.420 (6) | 0.635 (10) | 0.019* | |
O1 | 0.3992 (3) | 0.4115 (3) | 0.1817 (6) | 0.0167 (7) | |
H1O | 0.334 (6) | 0.400 (5) | 0.130 (10) | 0.020* | |
O2 | 0.4107 (3) | 0.2098 (3) | 0.1823 (6) | 0.0161 (7) | |
Br1 | 0.86980 (4) | 0.61815 (4) | −0.03325 (7) | 0.01281 (15) | |
Atomic displacement parameters (Å2) top | U11 | U22 | U33 | U12 | U13 | U23 |
C1 | 0.011 (2) | 0.009 (2) | 0.013 (2) | −0.0010 (16) | 0.0028 (17) | 0.0006 (16) |
C2 | 0.013 (2) | 0.009 (2) | 0.010 (2) | 0.0014 (16) | 0.0034 (16) | −0.0008 (16) |
C3 | 0.014 (2) | 0.005 (2) | 0.010 (2) | −0.0009 (16) | 0.0047 (17) | −0.0012 (16) |
C4 | 0.013 (2) | 0.012 (2) | 0.008 (2) | 0.0008 (17) | 0.0021 (16) | 0.0011 (16) |
C5 | 0.014 (2) | 0.011 (2) | 0.010 (2) | 0.0028 (17) | 0.0048 (17) | 0.0011 (17) |
C6 | 0.016 (2) | 0.009 (2) | 0.012 (2) | −0.0017 (16) | 0.0033 (18) | −0.0015 (17) |
C7 | 0.010 (2) | 0.011 (2) | 0.012 (2) | 0.0009 (16) | 0.0032 (17) | 0.0000 (17) |
N1 | 0.0112 (19) | 0.0086 (18) | 0.013 (2) | −0.0019 (15) | 0.0034 (16) | −0.0003 (16) |
N2 | 0.010 (2) | 0.015 (2) | 0.020 (2) | 0.0019 (17) | 0.0002 (16) | 0.0013 (17) |
O1 | 0.0083 (16) | 0.0094 (15) | 0.029 (2) | 0.0007 (12) | −0.0025 (14) | 0.0008 (13) |
O2 | 0.0118 (15) | 0.0097 (15) | 0.0247 (19) | −0.0020 (13) | −0.0001 (14) | −0.0012 (13) |
Br1 | 0.0108 (2) | 0.0136 (2) | 0.0130 (2) | −0.00047 (18) | 0.00053 (16) | −0.00011 (17) |
Geometric parameters (Å, º) top C1—C6 | 1.391 (6) | C5—H5 | 0.9500 |
C1—C2 | 1.400 (6) | C6—H6 | 0.9500 |
C1—C7 | 1.479 (6) | C7—O2 | 1.216 (5) |
C2—C3 | 1.379 (6) | C7—O1 | 1.326 (5) |
C2—H2 | 0.9500 | N1—H1N | 0.73 (6) |
C3—C4 | 1.398 (6) | N1—H2N | 0.90 (6) |
C3—N1 | 1.468 (5) | N1—H3N | 0.85 (6) |
C4—N2 | 1.387 (6) | N2—H4N | 0.81 (7) |
C4—C5 | 1.410 (6) | N2—H5N | 0.82 (6) |
C5—C6 | 1.383 (6) | O1—H1O | 0.79 (7) |
| | | |
C6—C1—C2 | 119.9 (4) | C5—C6—H6 | 119.9 |
C6—C1—C7 | 120.4 (4) | C1—C6—H6 | 119.9 |
C2—C1—C7 | 119.7 (4) | O2—C7—O1 | 123.2 (4) |
C3—C2—C1 | 119.1 (4) | O2—C7—C1 | 122.8 (4) |
C3—C2—H2 | 120.5 | O1—C7—C1 | 114.0 (4) |
C1—C2—H2 | 120.5 | C3—N1—H1N | 114 (5) |
C2—C3—C4 | 122.4 (4) | C3—N1—H2N | 107 (4) |
C2—C3—N1 | 118.1 (4) | H1N—N1—H2N | 99 (6) |
C4—C3—N1 | 119.5 (4) | C3—N1—H3N | 111 (4) |
N2—C4—C3 | 121.1 (4) | H1N—N1—H3N | 122 (6) |
N2—C4—C5 | 121.6 (4) | H2N—N1—H3N | 101 (5) |
C3—C4—C5 | 117.3 (4) | C4—N2—H4N | 110 (4) |
C6—C5—C4 | 120.9 (4) | C4—N2—H5N | 119 (5) |
C6—C5—H5 | 119.5 | H4N—N2—H5N | 111 (6) |
C4—C5—H5 | 119.5 | C7—O1—H1O | 111 (4) |
C5—C6—C1 | 120.3 (4) | | |
| | | |
C6—C1—C2—C3 | −0.7 (6) | C3—C4—C5—C6 | −2.1 (7) |
C7—C1—C2—C3 | −179.5 (4) | C4—C5—C6—C1 | 0.5 (7) |
C1—C2—C3—C4 | −1.0 (7) | C2—C1—C6—C5 | 1.0 (7) |
C1—C2—C3—N1 | 177.4 (4) | C7—C1—C6—C5 | 179.8 (4) |
C2—C3—C4—N2 | 179.9 (4) | C6—C1—C7—O2 | 0.4 (7) |
N1—C3—C4—N2 | 1.5 (7) | C2—C1—C7—O2 | 179.2 (4) |
C2—C3—C4—C5 | 2.4 (7) | C6—C1—C7—O1 | −179.9 (4) |
N1—C3—C4—C5 | −176.0 (4) | C2—C1—C7—O1 | −1.1 (6) |
N2—C4—C5—C6 | −179.6 (5) | | |
Hydrogen-bond geometry (Å, º) top D—H···A | D—H | H···A | D···A | D—H···A |
O1—H1O···Br1i | 0.79 (7) | 2.42 (7) | 3.199 (3) | 169 (6) |
N1—H1N···Br1 | 0.73 (6) | 2.70 (7) | 3.404 (5) | 163 (6) |
N1—H2N···O2ii | 0.90 (6) | 1.90 (6) | 2.787 (5) | 168 (5) |
N1—H3N···Br1iii | 0.85 (6) | 2.49 (6) | 3.333 (5) | 171 (5) |
N2—H4N···Br1iv | 0.81 (7) | 2.98 (7) | 3.705 (5) | 150 (5) |
N2—H5N···Br1v | 0.82 (6) | 2.98 (6) | 3.513 (4) | 125 (5) |
C2—H2···O2ii | 0.95 | 2.23 | 3.024 (5) | 140 |
Symmetry codes: (i) −x+1, −y+1, −z; (ii) −x+1, y+1/2, −z+1/2; (iii) x, y, z+1; (iv) −x+2, y−1/2, −z+1/2; (v) −x+2, −y+1, −z+1. |
2-Amino-5-carboxyanilinium nitrate monohydrate (III)
top Crystal data top C7H9N2O2+·NO3−·H2O | F(000) = 244 |
Mr = 233.19 | Dx = 1.630 Mg m−3 |
Monoclinic, P21 | Mo Kα radiation, λ = 0.71073 Å |
a = 3.8899 (3) Å | Cell parameters from 3653 reflections |
b = 9.8238 (6) Å | θ = 2.7–30.8° |
c = 12.5799 (9) Å | µ = 0.14 mm−1 |
β = 98.673 (7)° | T = 100 K |
V = 475.22 (6) Å3 | Slab, colourless |
Z = 2 | 0.20 × 0.10 × 0.05 mm |
Data collection top Rigaku Mercury CCD diffractometer | 2100 reflections with I > 2σ(I) |
ω scans | Rint = 0.067 |
Absorption correction: multi-scan (CrysAlisPRO; Rigaku, 2017) | θmax = 27.5°, θmin = 2.6° |
Tmin = 0.861, Tmax = 1.000 | h = −5→5 |
10770 measured reflections | k = −12→12 |
2157 independent reflections | l = −16→16 |
Refinement top Refinement on F2 | Primary atom site location: structure-invariant direct methods |
Least-squares matrix: full | Hydrogen site location: mixed |
R[F2 > 2σ(F2)] = 0.058 | H atoms treated by a mixture of independent and constrained refinement |
wR(F2) = 0.149 | w = 1/[σ2(Fo2) + (0.0747P)2 + 0.3934P] where P = (Fo2 + 2Fc2)/3 |
S = 1.15 | (Δ/σ)max = 0.005 |
2157 reflections | Δρmax = 0.48 e Å−3 |
155 parameters | Δρmin = −0.37 e Å−3 |
1 restraint | |
Special details top Geometry. All esds (except the esd in the dihedral angle between two l.s. planes) are estimated using the full covariance matrix. The cell esds are taken into account individually in the estimation of esds in distances, angles and torsion angles; correlations between esds in cell parameters are only used when they are defined by crystal symmetry. An approximate (isotropic) treatment of cell esds is used for estimating esds involving l.s. planes. |
Fractional atomic coordinates and isotropic or equivalent isotropic displacement parameters (Å2) top | x | y | z | Uiso*/Ueq | |
C1 | 0.2205 (11) | 0.5688 (5) | 0.4736 (3) | 0.0120 (8) | |
C2 | 0.2777 (10) | 0.4617 (5) | 0.4062 (3) | 0.0121 (8) | |
H2 | 0.4231 | 0.3880 | 0.4334 | 0.014* | |
C3 | 0.1240 (10) | 0.4617 (4) | 0.2997 (3) | 0.0120 (8) | |
C4 | −0.0999 (11) | 0.5677 (4) | 0.2573 (3) | 0.0124 (8) | |
C5 | −0.1462 (11) | 0.6769 (4) | 0.3255 (3) | 0.0128 (8) | |
H5 | −0.2878 | 0.7516 | 0.2982 | 0.015* | |
C6 | 0.0082 (11) | 0.6778 (4) | 0.4303 (3) | 0.0121 (8) | |
H6 | −0.0277 | 0.7530 | 0.4748 | 0.014* | |
C7 | 0.3867 (11) | 0.5736 (4) | 0.5864 (3) | 0.0127 (8) | |
N1 | 0.1979 (10) | 0.3534 (4) | 0.2274 (3) | 0.0137 (8) | |
H1N | −0.0055 | 0.3198 | 0.1919 | 0.016* | |
H2N | 0.3174 | 0.2856 | 0.2661 | 0.016* | |
H3N | 0.3279 | 0.3871 | 0.1790 | 0.016* | |
N2 | −0.2843 (11) | 0.5597 (4) | 0.1542 (3) | 0.0167 (8) | |
H4N | −0.198 (16) | 0.524 (6) | 0.097 (5) | 0.020* | |
H5N | −0.358 (16) | 0.637 (6) | 0.136 (4) | 0.020* | |
O1 | 0.5752 (9) | 0.4650 (3) | 0.6185 (2) | 0.0182 (7) | |
H1O | 0.685 (15) | 0.469 (7) | 0.685 (5) | 0.022* | |
O2 | 0.3588 (8) | 0.6720 (3) | 0.6453 (2) | 0.0156 (7) | |
N3 | 0.9275 (9) | 0.4263 (4) | 0.8918 (3) | 0.0147 (8) | |
O3 | 0.9624 (10) | 0.4968 (4) | 0.8102 (3) | 0.0234 (8) | |
O4 | 1.0903 (8) | 0.4593 (4) | 0.9805 (2) | 0.0210 (7) | |
O5 | 0.7311 (10) | 0.3252 (4) | 0.8821 (3) | 0.0263 (8) | |
O6 | 0.6514 (8) | 0.2329 (3) | 1.0947 (3) | 0.0181 (7) | |
H2O | 0.6684 | 0.2566 | 1.0297 | 0.022* | |
H3O | 0.6328 | 0.1351 | 1.0976 | 0.022* | |
Atomic displacement parameters (Å2) top | U11 | U22 | U33 | U12 | U13 | U23 |
C1 | 0.0129 (19) | 0.0080 (18) | 0.0155 (19) | −0.0052 (15) | 0.0033 (14) | 0.0015 (16) |
C2 | 0.0105 (17) | 0.0076 (18) | 0.019 (2) | 0.0003 (16) | 0.0059 (14) | 0.0019 (16) |
C3 | 0.0133 (19) | 0.0064 (18) | 0.0172 (19) | −0.0016 (17) | 0.0055 (14) | −0.0018 (16) |
C4 | 0.015 (2) | 0.0084 (19) | 0.015 (2) | 0.0014 (16) | 0.0058 (15) | 0.0003 (17) |
C5 | 0.015 (2) | 0.0044 (18) | 0.020 (2) | 0.0005 (16) | 0.0043 (15) | 0.0006 (17) |
C6 | 0.0125 (19) | 0.0046 (17) | 0.021 (2) | −0.0010 (15) | 0.0076 (15) | 0.0000 (16) |
C7 | 0.013 (2) | 0.0098 (19) | 0.015 (2) | −0.0043 (16) | 0.0020 (15) | 0.0000 (17) |
N1 | 0.0137 (17) | 0.0123 (18) | 0.0148 (17) | 0.0055 (14) | 0.0014 (13) | −0.0014 (14) |
N2 | 0.023 (2) | 0.0115 (18) | 0.0151 (18) | 0.0049 (15) | 0.0003 (14) | 0.0008 (15) |
O1 | 0.0250 (17) | 0.0146 (16) | 0.0134 (14) | 0.0011 (14) | −0.0017 (12) | 0.0004 (13) |
O2 | 0.0183 (16) | 0.0123 (15) | 0.0159 (14) | −0.0029 (12) | 0.0017 (11) | −0.0030 (13) |
N3 | 0.0165 (17) | 0.0137 (18) | 0.0137 (17) | 0.0016 (14) | 0.0021 (13) | −0.0029 (14) |
O3 | 0.0313 (19) | 0.0206 (17) | 0.0176 (16) | −0.0073 (14) | 0.0010 (13) | 0.0017 (14) |
O4 | 0.0226 (16) | 0.0222 (18) | 0.0176 (15) | −0.0014 (14) | 0.0012 (12) | −0.0029 (13) |
O5 | 0.032 (2) | 0.0202 (18) | 0.0268 (19) | −0.0136 (16) | 0.0056 (15) | −0.0046 (15) |
O6 | 0.0214 (16) | 0.0113 (14) | 0.0223 (16) | 0.0000 (13) | 0.0061 (13) | −0.0008 (12) |
Geometric parameters (Å, º) top C1—C2 | 1.391 (6) | C7—O1 | 1.323 (6) |
C1—C6 | 1.410 (6) | N1—H1N | 0.9100 |
C1—C7 | 1.469 (5) | N1—H2N | 0.9100 |
C2—C3 | 1.382 (5) | N1—H3N | 0.9100 |
C2—H2 | 0.9500 | N2—H4N | 0.91 (6) |
C3—C4 | 1.409 (6) | N2—H5N | 0.83 (6) |
C3—N1 | 1.457 (5) | O1—H1O | 0.88 (6) |
C4—N2 | 1.386 (5) | N3—O4 | 1.240 (5) |
C4—C5 | 1.402 (6) | N3—O5 | 1.247 (5) |
C5—C6 | 1.363 (6) | N3—O3 | 1.262 (5) |
C5—H5 | 0.9500 | O6—H2O | 0.8625 |
C6—H6 | 0.9500 | O6—H3O | 0.9644 |
C7—O2 | 1.232 (5) | | |
| | | |
C2—C1—C6 | 118.5 (4) | O2—C7—O1 | 123.0 (4) |
C2—C1—C7 | 121.7 (4) | O2—C7—C1 | 122.7 (4) |
C6—C1—C7 | 119.7 (4) | O1—C7—C1 | 114.3 (4) |
C3—C2—C1 | 120.3 (4) | C3—N1—H1N | 109.5 |
C3—C2—H2 | 119.8 | C3—N1—H2N | 109.5 |
C1—C2—H2 | 119.8 | H1N—N1—H2N | 109.5 |
C2—C3—C4 | 121.3 (4) | C3—N1—H3N | 109.5 |
C2—C3—N1 | 120.5 (4) | H1N—N1—H3N | 109.5 |
C4—C3—N1 | 118.2 (4) | H2N—N1—H3N | 109.5 |
N2—C4—C5 | 121.3 (4) | C4—N2—H4N | 124 (4) |
N2—C4—C3 | 121.0 (4) | C4—N2—H5N | 108 (4) |
C5—C4—C3 | 117.6 (4) | H4N—N2—H5N | 107 (5) |
C6—C5—C4 | 121.2 (4) | C7—O1—H1O | 115 (4) |
C6—C5—H5 | 119.4 | O4—N3—O5 | 121.2 (4) |
C4—C5—H5 | 119.4 | O4—N3—O3 | 119.0 (4) |
C5—C6—C1 | 121.0 (4) | O5—N3—O3 | 119.8 (4) |
C5—C6—H6 | 119.5 | H2O—O6—H3O | 108.8 |
C1—C6—H6 | 119.5 | | |
| | | |
C6—C1—C2—C3 | −1.1 (6) | C3—C4—C5—C6 | −2.7 (6) |
C7—C1—C2—C3 | −178.4 (4) | C4—C5—C6—C1 | 0.1 (6) |
C1—C2—C3—C4 | −1.6 (6) | C2—C1—C6—C5 | 1.8 (6) |
C1—C2—C3—N1 | 176.7 (4) | C7—C1—C6—C5 | 179.2 (4) |
C2—C3—C4—N2 | −172.5 (4) | C2—C1—C7—O2 | 174.8 (4) |
N1—C3—C4—N2 | 9.2 (6) | C6—C1—C7—O2 | −2.5 (6) |
C2—C3—C4—C5 | 3.4 (6) | C2—C1—C7—O1 | −3.7 (6) |
N1—C3—C4—C5 | −174.9 (4) | C6—C1—C7—O1 | 179.0 (4) |
N2—C4—C5—C6 | 173.3 (4) | | |
Hydrogen-bond geometry (Å, º) top D—H···A | D—H | H···A | D···A | D—H···A |
N1—H1N···O6i | 0.91 | 1.87 | 2.764 (5) | 165 |
N1—H2N···O2ii | 0.91 | 1.91 | 2.808 (5) | 168 |
N1—H3N···N2iii | 0.91 | 2.32 | 3.094 (6) | 143 |
N1—H3N···O6iv | 0.91 | 2.33 | 2.862 (5) | 118 |
N2—H4N···O4i | 0.91 (6) | 2.07 (6) | 2.972 (5) | 169 (5) |
N2—H5N···O5v | 0.83 (6) | 2.34 (7) | 3.129 (5) | 159 (6) |
O1—H1O···O3 | 0.88 (6) | 1.79 (6) | 2.662 (5) | 170 (6) |
O6—H2O···O5 | 0.86 | 2.02 | 2.884 (5) | 176 |
O6—H3O···O3vi | 0.96 | 2.26 | 2.920 (5) | 125 |
O6—H3O···O4vi | 0.96 | 2.33 | 3.068 (5) | 133 |
C2—H2···O2ii | 0.95 | 2.54 | 3.285 (5) | 135 |
Symmetry codes: (i) x−1, y, z−1; (ii) −x+1, y−1/2, −z+1; (iii) x+1, y, z; (iv) x, y, z−1; (v) −x, y+1/2, −z+1; (vi) −x+2, y−1/2, −z+2. |
Aromatic π–π stacking interactions in the title compounds topAll interactions involve the C1–C6 benzene rings. Cg···Cg is the centroid–centroid separation, α is the dihedral angle between the ring planes. |
Compound | Cg···Cg (Å) | α (°) | slippage (Å) | symmetry |
(I) | 3.8895 (9) | 1.79 (7) | 1.902 | x, 1 - y, z - 1/2 |
(I) | 3.8895 (9) | 1.79 (7) | 1.822 | x, 1 - y, z + 1/2 |
(II) | 3.736 (3) | 1.5 (2) | 2.035 | x, 1/2 - y, z - 1/2 |
(II) | 3.736 (3) | 1.5 (2) | 1.954 | x, 1/2 - y, z + 1/2 |
(III) | 3.890 (2) | 0.0 (2) | 2.105 | x - 1, y, z |
(III) | 3.890 (2) | 0.0 (2) | 2.105 | x + 1, y, z |
Hirshfeld fingerprint contact percentages for different intermolecular interactions in the title compounds topInteraction | (I) | (II) | (III) |
H···H | 30.3 | 30.7 | 23.3 |
H···Xa | 14.5 | 15.2 | – |
H···O (donor) | 10.6 | 10.0 | 32.2 |
H···C | 6.8 | 6.7 | 5.8 |
H···N | 1.9 | 2.2 | 2.3 |
C···C | 6.7 | 6.8 | 6.4 |
C···H | 8.6 | 8.6 | 9.0 |
C···O | 2.2 | 2.4 | 2.9 |
N···H | 2.1 | 2.7 | 2.1 |
O···H (acceptor) | 13.3 | 11.6 | 12.6 |
Xa···H | 99.8 | 98.7 | – |
Note: (a) For (I), X = Cl; for (II) X = Br. |
Acknowledgements
We thank the EPSRC National Crystallography Service (University of Southampton) for the intensity data collections.
References
Davies, L. H., Kasten, B. B., Benny, P. D., Arrowsmith, R. L., Ge, H., Pascu, S. I., Botchway, S. W., Clegg, W., Harrington, R. W. & Higham, L. J. (2014). Chem. Commun. 50, 15503–15505. Web of Science CSD CrossRef CAS Google Scholar
Farrugia, L. J. (2012). J. Appl. Cryst. 45, 849–854. Web of Science CrossRef CAS IUCr Journals Google Scholar
Fernández-Palacio, F., Restrepo, J., Gálvez, S., Gómez-Sal, P. & Mosquera, M. E. G. (2014). CrystEngComm, 16, 3376–3386. Google Scholar
Groom, C. R., Bruno, I. J., Lightfoot, M. P. & Ward, S. C. (2016). Acta Cryst. B72, 171–179. Web of Science CrossRef IUCr Journals Google Scholar
Khosa, M. K., Wood, P. T., Humphreys, S. M. & Harrison, W. T. A. (2015). J. Struct. Chem. 56, 1130–1135. Web of Science CSD CrossRef CAS Google Scholar
Lai, T. F. & Marsh, R. E. (1967). Acta Cryst. 22, 885–893. CSD CrossRef CAS IUCr Journals Web of Science Google Scholar
Pruchnik, F. P., Bańbuła, M., Ciunik, Z., Chojnacki, H., Latocha, M., Skop, B., Wilczok, T., Opolski, A., Wietrzyk, J. & Nasulewicz, A. (2002). Eur. J. Inorg. Chem. pp. 3214–3221. CrossRef Google Scholar
Rigaku (2017). CrysAlis PRO. Rigaku Inc., Tokyo, Japan. Google Scholar
Rzaczyńska, Z., Bartyzel, A. & Głowiak, T. (2003). J. Coord. Chem. 56, 77–83. Google Scholar
Rzaczyńska, Z., Belskii, V. K. & Zavodnik, V. E. (1994). Polish J. Chem. 68, 1639–1647. Google Scholar
Rzaczyńska, Z., Mrozek, R., Lenik, J., Sikorska, M. & Głowiak, T. (2000). J. Chem. Crystallogr. 30, 519–524. Google Scholar
Sheldrick, G. M. (2008). Acta Cryst. A64, 112–122. Web of Science CrossRef CAS IUCr Journals Google Scholar
Sheldrick, G. M. (2015). Acta Cryst. C71, 3–8. Web of Science CrossRef IUCr Journals Google Scholar
Tan, S. L., Jotani, M. M. & Tiekink, E. R. T. (2019). Acta Cryst. E75, 308–318. Web of Science CrossRef IUCr Journals Google Scholar
Turner, M. J., McKinnon, J. J., Wolff, S. K., Grimwood, D. J., Spackman, P. R., Jayatilaka, D. & Spackman, M. A. (2017). CrystalExplorer17. University of Western Australia. https://hirshfeldsurface.net. Google Scholar
Westrip, S. P. (2010). J. Appl. Cryst. 43, 920–925. Web of Science CrossRef CAS IUCr Journals Google Scholar
This is an open-access article distributed under the terms of the Creative Commons Attribution (CC-BY) Licence, which permits unrestricted use, distribution, and reproduction in any medium, provided the original authors and source are cited.
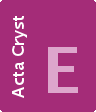 | CRYSTALLOGRAPHIC COMMUNICATIONS |
ISSN: 2056-9890
Open

access