1. Chemical context
The recently published structure of 3-chloro-N,N-dimethylpropan-1-aminium chloride reported the conformation of the molecular cation (henceforth 3CLPA+) as gauche for the terminal Cl atom (Bond & Silwal, 2023
). DFT geometry optimizations in vacuo indicate that the gauche conformation is more stable than anti for the molecular cation, as well as for the 2-chloro-N,N-dimethylethan-1-aminium analog (henceforth 2CLEA+). In both cases the molecular cations appear to exhibit the gauche effect in which the bonding pair of a C—H bond β to the terminal halogen atom of the chain is donated to the antibonding orbital of the C—X bond (X = halogen) to stabilize the gauche conformation through hyperconjugation (Wolfe, 1972
). A recent computational study of 1,2-dihaloethanes reports that this stabilization due to hyperconjugation is always present, but other energy contributions – such as steric interactions – are more important so that the gauche effect is typically observed only with fluorine (Rodrigues Silva et al., 2021
). However, in the 3CLPA+ and 2CLEA+ cations the terminal Cl atom is closer to the formal center of positive charge when in the gauche conformation, which may provide an additional contribution to energetic stability.
We are interested in investigating the competition between gauche and anti conformations for these molecular cations in different chemical environments in order to explore their possible use as molecular switches. If the gauche conformation is stabilized by interaction with the positive charge center in the cation, then it is possible with loss of this interaction through deprotonation that the anti conformation becomes more stable and would allow for a change in conformation by altering the degree of protonation. Here we report the structures of 2CLEA+ with the tetrahedral complex anions CoCl42− and ZnCl42−.
2. Structural commentary
The structures are isomorphous with unit-cell parameters in close agreement, e.g. unit-cell volumes agree within 2 s.u. Both tetrahedral complexes occupy sites of twofold rotational symmetry with a slight flattening about the twofold axis to produce some Cl—M—Cl (M = Co, Zn) angles greater than 109.5°. M—Cl bond lengths range from 2.25–2.29 Å, in good agreement with average bond lengths of 2.27 (2) Å for CoCl42− and 2.27 (4) Å for ZnCl42− calculated from structures in the Cambridge Structural Database [512 and 960 hits, respectively; version 5.45 (November, 2023); Groom et al. (2016
)].
Of greater interest is the organic cation, which exhibits disorder between the gauche [s.o.f = 0.707 (2) for CoCl42− and 0.697 (2) for ZnCl42−] and anti conformations (the dimethylammonium head group is ordered). The N1—C1—C2—Cl3 torsion angles are 61.6 (7)° in the CoCl42− and −61.3 (6)° in the ZnCl42− salts for the gauche conformation and, likewise, −179.7 (13) and 179.3 (9)°, respectively, for the anti. Bond lengths and angles within the cation correspond to expected values, disregarding small distortions that arise due to refinement of atoms of the disordered pair in close proximity. Displacement ellipsoid plots with labels for non-H atoms are presented in Fig. 1
for the CoCl42− salt showing only the gauche conformation of the organic cation, and in Fig. 2
for the ZnCl42− salt showing only the anti conformation. Bond lengths and angles for non-H atoms are presented in Table 1
for the CoCl42− salt, with only the gauche conformation values, and in Table 2
for the ZnCl42− salt, with only the anti conformation values.
Co1—Cl1 | 2.2873 (6) | N1—C1 | 1.509 (5) | Co1—Cl2 | 2.2618 (6) | C1—C2 | 1.534 (6) | N1—C3 | 1.482 (3) | C2—Cl3 | 1.776 (3) | N1—C4 | 1.483 (3) | | | | | | | Cl1i—Co1—Cl1 | 111.86 (3) | C3—N1—C1 | 105.4 (3) | Cl1—Co1—Cl2 | 108.17 (2) | C4—N1—C1 | 114.8 (4) | Cl1—Co1—Cl2i | 104.58 (2) | N1—C1—C2 | 116.2 (5) | Cl2i—Co1—Cl2 | 119.62 (4) | C1—C2—Cl3 | 111.0 (4) | C3—N1—C4 | 110.6 (2) | | | Symmetry code: (i) . | |
Zn1—Cl2 | 2.2553 (5) | N1—C4 | 1.485 (3) | Zn1—Cl1 | 2.2883 (5) | N1—C1 | 1.515 (5) | N1—C1A | 1.476 (9) | C1—C2 | 1.535 (5) | N1—C3 | 1.480 (3) | C2—Cl3 | 1.779 (3) | | | | | Cl2—Zn1—Cl2i | 118.93 (3) | C3—N1—C4 | 110.5 (2) | Cl2—Zn1—Cl1i | 104.94 (2) | C3—N1—C1 | 105.3 (2) | Cl2—Zn1—Cl1 | 108.36 (2) | C4—N1—C1 | 114.6 (3) | Cl1i—Zn1—Cl1 | 111.38 (3) | N1—C1—C2 | 116.3 (4) | C1A—N1—C3 | 119.8 (5) | C1—C2—Cl3 | 110.7 (3) | C1A—N1—C4 | 111.1 (8) | | | Symmetry code: (i) . | |
| Figure 1 Displacement ellipsoid plot (50% level) of the organic cation and complex anion in (2CLEA+)2CoCl4 with labels for non-H atoms. The gauche conformation of the organic cation only is shown. H atoms are drawn as circles of arbitrary radii and N—H⋯Cl hydrogen bonding is represented by dashed lines. |
| Figure 2 Displacement ellipsoid plot (50% level) of the organic cation and complex anion in (2CLEA+)2ZnCl4 with labels for non-H atoms. The anti conformation of the organic cation only is shown. H atoms are drawn as circles of arbitrary radii and N—H⋯Cl hydrogen bonding is represented by dashed lines. |
DFT geometry optimizations [B3LYP, 6311+G(d,p); GAMESS (Schmidt et al., 1993
)] in vacuo of 2CLEA+ yield an energy for the gauche conformation that is 0.226 eV less than the anti conformation for a 52.737° N—C—C—Cl torsion angle (0.228 eV less for a torsion angle of −52.738°). To approximate the ionic environment of the cation in the crystal, the optimizations were performed in a uniform dielectric constant of 78.4. This results in a reduction of gauche conformation stabilization to 0.0584 eV (torsion angle = 59.1°; by 0.0582 eV for torsion angle = −58.785°), but yields better agreement with observed torsion angle values. [Optimized torsion angles for the anti conformation with magnitudes of 173.858° (in vacuo) and 173.819° (dielectric) deviate slightly from observed values.] Similar optimizations for the unprotonated molecule show both gauche conformations are destabilized in vacuo (by 0.0412 eV for torsion angle = 66.377° and by 0.0428 eV for torsion angle = −64.735°). Energy differences for optimizations performed in uniform dielectric for the unprotonated molecule are not as stark: gauche conformations are slightly stabilized by 0.00792 eV (−65.149°) or 0.00624 eV (64.459°). These results show promise of a switch from gauche to anti via deprotonation. An electrostatic potential plot of the gauche conformation from the uniform dielectric calculation is presented in Fig. 3
.
| Figure 3 Electrostatic potential plot of the 2CLEA+ cation calculated in uniform dielectric for the gauche conformation with a ball-and-stick model of the optimized geometry shown within the electron density envelope. Red indicates regions of negative charge accumulation and blue regions of positive charge. |
3. Supramolecular features
The dimethylammonium headgroup forms an asymmetric, bifurcated hydrogen bond to Cl1 and Cl2 resulting in the most acute Cl—M—Cl angle in the complex anion – and a likely origin for the observed tetrahedral flattening. Hydrogen-bond lengths and angles are presented in Tables 3
and 4
for the CoCl42− and ZnCl42− salts, respectively.
D—H⋯A | D—H | H⋯A | D⋯A | D—H⋯A | N1—H1⋯Cl1 | 0.87 (3) | 2.51 (3) | 3.3093 (19) | 152 (2) | N1—H1⋯Cl2i | 0.87 (3) | 3.02 (3) | 3.564 (2) | 122 (2) | Symmetry code: (i) . | |
D—H⋯A | D—H | H⋯A | D⋯A | D—H⋯A | N1—H1⋯Cl1i | 0.87 (3) | 2.51 (3) | 3.3124 (18) | 156 (2) | N1—H1⋯Cl2 | 0.87 (3) | 3.03 (3) | 3.5620 (19) | 121 (2) | Symmetry code: (i) . | |
In the gauche conformation of the molecular cation, the terminal Cl atom is placed slightly offset from the center of the tetrahedral face defined by Cl1, Cl2, and Cl2i [symmetry code: (i) 1 − x, y,
− z]. This places the terminal Cl atom at a distance of 3.7576 (9) Å from the Co2+ center [3.7690 (10) Å for Zn2+] with the shortest Cl⋯Cl contact distance [Cl2⋯Cl3 = 3.4293 (11) Å for CoCl42−; 3.4237 (11) Å for ZnCl42−] slightly less than 3.50 Å – the sum of the van der Waals radii. In the anti conformation, a methylene H atom from the carbon α to the terminal Cl atom is instead directed at this face and forms contact distances of 3.2–3.3 Å to the Cl atoms. Meanwhile, the terminal Cl atom now forms a short contact [Cl3A⋯Cl3Aii = 2.588 (4) Å, Cl3A⋯Cl3Aiii = 2.568 (4) Å; symmetry codes: (ii)
− x,
− y, 1 − z, for CoCl42−; (iii)
− x,
− y, −z, for ZnCl42−] with a terminal anti conformation Cl atom in the nearest neighbor that is ∼1 Å less than the sum of the van der Waals radii. Hence, any anti conformation molecular cation must have a gauche conformation cation as a nearest neighbor. This provides another driver for the dominance of the gauche conformation in these structures.
The three-dimensional packing can be described starting with parallel rows of hydrogen-bonded formula units along (101) arranged into layers in the ac plane, as shown in the layer packing diagram of Fig. 4
. Rows in neighboring layers nest between the rows of a given layer, with neighboring layers related by the C-centering translation, as shown in the unit-cell packing diagram of Fig. 5
.
| Figure 4 Layer packing diagram viewed down the b axis for (2CLEA+)2CoCl4 (gauche conformation only) that depicts portions of two rows of formula units along (101) that form a layer in the ac plane. The a axis slants down and to the right, the c axis slants up and to the right. Atoms are drawn as circles of arbitrary radii and N—H⋯Cl hydrogen bonding is represented by dashed lines |
| Figure 5 Unit-cell packing diagram for (2CLEA+)2ZnCl4 viewed down (101) showing the stacking of four of the layers presented in Fig. 4 with the b axis vertical. H atoms are omitted for clarity (except for N—H), atoms are drawn as circles of arbitrary radii, and N—H⋯Cl hydrogen bonding is represented by dashed lines. |
4. Database survey
Structural results for 2CLEA+ or 3CLPA+ cations have been sparsely reported. A survey of the Cambridge Structural Database (version 5.45, November, 2023; Groom et al., 2016
). yields only two known prior examples of structures containing 2CLEA+: an (Mo2O2Cl8)2− salt (CSD refcode POSWAX; Marchetti et al., 2015
), in which the gauche conformation is found, and a chloride salt (CSD refcode: URORUR; Muller et al., 2021
) where the anti conformation is found, albeit with a disordered alkyl chain. For 3CLPA+, besides the aforementioned chloride salt there is one other structure containing 3-chloro-N,N-propan-1-amine as a ligand in a di(μ-hydrido) dialuminium complex (CSD refcode: NIGGOZ; Andrews et al., 1997
) where the gauche conformation is also found. No structures containing the unprotonated or uncoordinated molecules have been reported. There are also no reported structures for the longer chain chlorobutyl or chloropentyl analogs.
5. Synthesis and crystallization
Both compounds were prepared by dissolving 2-chloro-N,N-dimethylethan-1-aminium chloride with 1.00 g of CoCl2·6H2O or ZnCl2 in a 2:1 molar ratio in water. The solutions were acidified with concentrated HCl(aq) to yield ∼6 M HCl and produce a definite blue color in the cobalt(II) solution. The solutions were evaporated to a syrup with the syrup redissolved in ethanol to yield crystals of the title compounds upon further evaporation.
6. Refinement
Crystal data, data collection, and structure refinement details are summarized in Table 5
. For both compounds, initial structure solution identified positions of all non-H atoms except those of the anti conformation. Prominent electron density difference map peaks then identified atoms of the anti conformation. Common site occupation factors for each conformation were refined with the constraint that their sum equal 1.0. H-atom positions were visible on the electron density difference map, but were calculated and refined using a riding model for those bound to C with isotropic displacement parameters set to 1.2 or 1.5×Uiso of the parent atom for methylene or methyl H atoms, respectively. The H atom bound to N was freely refined to a reasonable N—H bond length and the N1—C1A distance was constrained to a chemically reasonable distance (1.50±0.01 Å) using the DFIX command in SHELX.
| Cobaltate | Zincate | Crystal data | Chemical formula | (C4H11ClN)2[CoCl4] | (C4H11ClN)2[ZnCl4] | Mr | 417.93 | 424.34 | Crystal system, space group | Monoclinic, C2/c | Monoclinic, C2/c | Temperature (K) | 295 | 295 | a, b, c (Å) | 12.7521 (6), 8.9648 (4), 16.6801 (10) | 12.7297 (7), 8.9784 (5), 16.6837 (11) | β (°) | 111.057 (1) | 111.062 (2) | V (Å3) | 1779.53 (16) | 1779.43 (18) | Z | 4 | 4 | Radiation type | Mo Kα | Mo Kα | μ (mm−1) | 1.85 | 2.26 | Crystal size (mm) | 0.30 × 0.27 × 0.26 | 0.39 × 0.38 × 0.29 | | Data collection | Diffractometer | Bruker D8 Quest Eco | Bruker D8 Quest Eco | Absorption correction | Multi-scan (SADABS; Krause et al., 2015 ) | Multi-scan (SADABS; Krause et al., 2015 ) | Tmin, Tmax | 0.696, 0.746 | 0.472, 0.560 | No. of measured, independent and observed [I > 2σ(I)] reflections | 23748, 2041, 1676 | 46381, 2843, 2479 | Rint | 0.042 | 0.036 | (sin θ/λ)max (Å−1) | 0.650 | 0.725 | | Refinement | R[F2 > 2σ(F2)], wR(F2), S | 0.030, 0.064, 1.09 | 0.033, 0.070, 1.12 | No. of reflections | 2041 | 2843 | No. of parameters | 113 | 113 | No. of restraints | 1 | 1 | H-atom treatment | H atoms treated by a mixture of independent and constrained refinement | H atoms treated by a mixture of independent and constrained refinement | Δρmax, Δρmin (e Å−3) | 0.38, −0.29 | 0.57, −0.40 | Computer programs: APEX3 and SAINT (Bruker, 2017 ), SHELXT2018/2 (Sheldrick, 2015a ), SHELXL2018/3 (Sheldrick 2015b ), ORTEP-3 for Windows (Farrugia, 2012 ) and publCIF (Westrip, 2010 ). | |
Low angle reflections (four for CoCl42− and one for ZnCl42− salts) with Fo2<<Fc2 were assumed to be blocked by the beam catcher and were omitted from the refinement. For the ZnCl42− structure, APEX3 control software suggested a data-collection strategy to θmax = 36°. However data analysis (WinGX 2021.3; Farrugia, 2012
) indicated <I/σ> less than 2.0 for reflections beyond θ = 30.6°. Thus reflection data beyond θ = 31° were omitted from the final refinement.
Supporting information
Bis(2-chloro-
N,
N-dimethylethan-1-aminium) tetrachloridocobaltate(II) (cobaltate)
top Crystal data top (C4H11ClN)2[CoCl4] | F(000) = 852 |
Mr = 417.93 | Dx = 1.56 Mg m−3 |
Monoclinic, C2/c | Mo Kα radiation, λ = 0.71073 Å |
Hall symbol: -C 2yc | Cell parameters from 9986 reflections |
a = 12.7521 (6) Å | θ = 2.9–27.4° |
b = 8.9648 (4) Å | µ = 1.85 mm−1 |
c = 16.6801 (10) Å | T = 295 K |
β = 111.057 (1)° | Gem, blue |
V = 1779.53 (16) Å3 | 0.30 × 0.27 × 0.26 mm |
Z = 4 | |
Data collection top Bruker D8 Quest Eco diffractometer | 1676 reflections with I > 2σ(I) |
φ and ω scans | Rint = 0.042 |
Absorption correction: multi-scan (SADABS; Krause et al., 2015) | θmax = 27.5°, θmin = 3.4° |
Tmin = 0.696, Tmax = 0.746 | h = −16→16 |
23748 measured reflections | k = −11→11 |
2041 independent reflections | l = −21→21 |
Refinement top Refinement on F2 | Hydrogen site location: mixed |
Least-squares matrix: full | H atoms treated by a mixture of independent and constrained refinement |
R[F2 > 2σ(F2)] = 0.030 | w = 1/[σ2(Fo2) + (0.0202P)2 + 2.2595P] where P = (Fo2 + 2Fc2)/3 |
wR(F2) = 0.064 | (Δ/σ)max = 0.001 |
S = 1.09 | Δρmax = 0.38 e Å−3 |
2041 reflections | Δρmin = −0.29 e Å−3 |
113 parameters | Extinction correction: SHELXL2018/3 (Sheldrick 2015b) |
1 restraint | Extinction coefficient: 0.0038 (3) |
Primary atom site location: dual | |
Special details top Geometry. All esds (except the esd in the dihedral angle between two l.s. planes) are estimated using the full covariance matrix. The cell esds are taken into account individually in the estimation of esds in distances, angles and torsion angles; correlations between esds in cell parameters are only used when they are defined by crystal symmetry. An approximate (isotropic) treatment of cell esds is used for estimating esds involving l.s. planes. |
Fractional atomic coordinates and isotropic or equivalent isotropic displacement parameters (Å2) top | x | y | z | Uiso*/Ueq | Occ. (<1) |
Co1 | 0.5 | 0.77754 (4) | 0.25 | 0.03399 (13) | |
Cl1 | 0.63314 (4) | 0.92047 (6) | 0.34886 (3) | 0.04269 (15) | |
Cl2 | 0.40423 (5) | 0.65066 (7) | 0.31892 (4) | 0.05376 (18) | |
N1 | 0.84438 (16) | 0.6926 (2) | 0.36634 (12) | 0.0402 (4) | |
H1 | 0.775 (2) | 0.725 (3) | 0.3517 (17) | 0.066 (8)* | |
C1 | 0.8515 (7) | 0.5245 (5) | 0.3673 (6) | 0.0395 (13) | 0.707 (2) |
H1A | 0.929586 | 0.496545 | 0.381431 | 0.047* | 0.707 (2) |
H1B | 0.810217 | 0.488369 | 0.309775 | 0.047* | 0.707 (2) |
C2 | 0.8069 (3) | 0.4440 (4) | 0.4297 (2) | 0.0465 (8) | 0.707 (2) |
H2A | 0.848364 | 0.477204 | 0.487909 | 0.056* | 0.707 (2) |
H2B | 0.818475 | 0.337488 | 0.427118 | 0.056* | 0.707 (2) |
Cl3 | 0.66156 (8) | 0.48055 (11) | 0.40385 (6) | 0.0581 (3) | 0.707 (2) |
C1A | 0.8273 (19) | 0.5310 (12) | 0.3788 (17) | 0.055 (5) | 0.293 (2) |
H1AA | 0.800336 | 0.479291 | 0.324113 | 0.066* | 0.293 (2) |
H1AB | 0.897006 | 0.48509 | 0.415285 | 0.066* | 0.293 (2) |
C2A | 0.7424 (9) | 0.5258 (10) | 0.4203 (6) | 0.051 (2) | 0.293 (2) |
H2AA | 0.672082 | 0.570241 | 0.383851 | 0.061* | 0.293 (2) |
H2AB | 0.768918 | 0.576965 | 0.475201 | 0.061* | 0.293 (2) |
Cl3A | 0.7270 (3) | 0.3331 (3) | 0.43356 (19) | 0.0799 (11) | 0.293 (2) |
C3 | 0.8885 (2) | 0.7413 (3) | 0.29966 (17) | 0.0572 (7) | |
H3A | 0.885462 | 0.848115 | 0.295397 | 0.086* | |
H3B | 0.843773 | 0.698591 | 0.245341 | 0.086* | |
H3C | 0.964987 | 0.708767 | 0.315027 | 0.086* | |
C4 | 0.9070 (3) | 0.7654 (3) | 0.44973 (17) | 0.0687 (8) | |
H4A | 0.984779 | 0.737603 | 0.468255 | 0.103* | |
H4B | 0.876356 | 0.733971 | 0.491816 | 0.103* | |
H4C | 0.900302 | 0.871732 | 0.443083 | 0.103* | |
Atomic displacement parameters (Å2) top | U11 | U22 | U33 | U12 | U13 | U23 |
Co1 | 0.0301 (2) | 0.0362 (2) | 0.0354 (2) | 0 | 0.01147 (16) | 0 |
Cl1 | 0.0383 (3) | 0.0475 (3) | 0.0375 (3) | −0.0055 (2) | 0.0078 (2) | −0.0033 (2) |
Cl2 | 0.0611 (4) | 0.0447 (3) | 0.0666 (4) | −0.0097 (3) | 0.0364 (3) | 0.0013 (3) |
N1 | 0.0339 (9) | 0.0456 (11) | 0.0413 (10) | 0.0079 (8) | 0.0137 (8) | −0.0021 (8) |
C1 | 0.043 (4) | 0.040 (2) | 0.042 (3) | −0.0033 (16) | 0.023 (2) | 0.0027 (16) |
C2 | 0.0492 (19) | 0.0449 (19) | 0.0467 (19) | 0.0064 (16) | 0.0188 (15) | 0.0019 (16) |
Cl3 | 0.0479 (6) | 0.0665 (6) | 0.0663 (6) | −0.0018 (4) | 0.0280 (4) | −0.0007 (5) |
C1A | 0.038 (9) | 0.086 (10) | 0.047 (9) | −0.015 (6) | 0.024 (5) | 0.001 (6) |
C2A | 0.062 (6) | 0.047 (5) | 0.048 (5) | 0.004 (5) | 0.025 (4) | 0.009 (4) |
Cl3A | 0.113 (2) | 0.0584 (16) | 0.0850 (19) | −0.0153 (14) | 0.0554 (17) | 0.0128 (13) |
C3 | 0.0704 (17) | 0.0520 (15) | 0.0552 (15) | −0.0085 (13) | 0.0297 (13) | 0.0012 (12) |
C4 | 0.081 (2) | 0.0703 (19) | 0.0485 (15) | 0.0016 (15) | 0.0150 (14) | −0.0161 (14) |
Geometric parameters (Å, º) top Co1—Cl1 | 2.2873 (6) | C1—H1B | 0.97 |
Co1—Cl2 | 2.2618 (6) | C1—C2 | 1.534 (6) |
N1—C3 | 1.482 (3) | C2—H2A | 0.97 |
C3—H3A | 0.96 | C2—H2B | 0.97 |
C3—H3B | 0.96 | C2—Cl3 | 1.776 (3) |
C3—H3C | 0.96 | N1—C1A | 1.490 (10) |
N1—C4 | 1.483 (3) | C1A—H1AA | 0.97 |
C4—H4A | 0.96 | C1A—H1AB | 0.97 |
C4—H4B | 0.96 | C1A—C2A | 1.480 (15) |
C4—H4C | 0.96 | C2A—H2AA | 0.97 |
N1—H1 | 0.87 (3) | C2A—H2AB | 0.97 |
N1—C1 | 1.509 (5) | C2A—Cl3A | 1.762 (9) |
C1—H1A | 0.97 | | |
| | | |
Cl1i—Co1—Cl1 | 111.86 (3) | C2—C1—H1A | 108.2 |
Cl1—Co1—Cl2 | 108.17 (2) | N1—C1—H1B | 108.2 |
Cl1—Co1—Cl2i | 104.58 (2) | C2—C1—H1B | 108.2 |
Cl2i—Co1—Cl2 | 119.62 (4) | H1A—C1—H1B | 107.4 |
C3—N1—C4 | 110.6 (2) | C1—C2—Cl3 | 111.0 (4) |
N1—C3—H3A | 109.5 | C1—C2—H2A | 109.4 |
N1—C3—H3B | 109.5 | Cl3—C2—H2A | 109.4 |
H3A—C3—H3B | 109.5 | C1—C2—H2B | 109.4 |
N1—C3—H3C | 109.5 | Cl3—C2—H2B | 109.4 |
H3A—C3—H3C | 109.5 | H2A—C2—H2B | 108 |
H3B—C3—H3C | 109.5 | C3—N1—C1A | 120.4 (7) |
N1—C4—H4A | 109.5 | C4—N1—C1A | 110.7 (11) |
N1—C4—H4B | 109.5 | C2A—C1A—N1 | 105.3 (9) |
H4A—C4—H4B | 109.5 | C2A—C1A—H1AA | 110.7 |
N1—C4—H4C | 109.5 | N1—C1A—H1AA | 110.7 |
H4A—C4—H4C | 109.5 | C2A—C1A—H1AB | 110.7 |
H4B—C4—H4C | 109.5 | N1—C1A—H1AB | 110.7 |
C3—N1—C1 | 105.4 (3) | H1AA—C1A—H1AB | 108.8 |
C4—N1—C1 | 114.8 (4) | C1A—C2A—Cl3A | 102.9 (7) |
C3—N1—H1 | 108.0 (18) | C1A—C2A—H2AA | 111.2 |
C4—N1—H1 | 105.3 (18) | Cl3A—C2A—H2AA | 111.2 |
C1A—N1—H1 | 100 (2) | C1A—C2A—H2AB | 111.2 |
C1—N1—H1 | 112.6 (19) | Cl3A—C2A—H2AB | 111.2 |
N1—C1—C2 | 116.2 (5) | H2AA—C2A—H2AB | 109.1 |
N1—C1—H1A | 108.2 | | |
| | | |
C3—N1—C1—C2 | −178.1 (5) | C3—N1—C1A—C2A | −156.9 (10) |
C4—N1—C1—C2 | 59.9 (7) | C4—N1—C1A—C2A | 71.9 (16) |
N1—C1—C2—Cl3 | 61.6 (7) | N1—C1A—C2A—Cl3A | −179.7 (13) |
Symmetry code: (i) −x+1, y, −z+1/2. |
Hydrogen-bond geometry (Å, º) top D—H···A | D—H | H···A | D···A | D—H···A |
N1—H1···Cl1 | 0.87 (3) | 2.51 (3) | 3.3093 (19) | 152 (2) |
N1—H1···Cl2i | 0.87 (3) | 3.02 (3) | 3.564 (2) | 122 (2) |
Symmetry code: (i) −x+1, y, −z+1/2. |
Bis(2-chloro-
N,
N-dimethylethan-1-aminium) tetrachloridozincate(II) (zincate)
top Crystal data top (C4H11ClN)2[ZnCl4] | F(000) = 864 |
Mr = 424.34 | Dx = 1.584 Mg m−3 |
Monoclinic, C2/c | Mo Kα radiation, λ = 0.71073 Å |
Hall symbol: -C 2yc | Cell parameters from 9979 reflections |
a = 12.7297 (7) Å | θ = 2.8–33.7° |
b = 8.9784 (5) Å | µ = 2.26 mm−1 |
c = 16.6837 (11) Å | T = 295 K |
β = 111.062 (2)° | Irregular, colourless |
V = 1779.43 (18) Å3 | 0.39 × 0.38 × 0.29 mm |
Z = 4 | |
Data collection top Bruker D8 Quest Eco diffractometer | 2479 reflections with I > 2σ(I) |
φ and ω scans | Rint = 0.036 |
Absorption correction: multi-scan (SADABS; Krause et al., 2015) | θmax = 31.0°, θmin = 3.4° |
Tmin = 0.472, Tmax = 0.560 | h = −18→18 |
46381 measured reflections | k = −12→12 |
2843 independent reflections | l = −24→24 |
Refinement top Refinement on F2 | Hydrogen site location: mixed |
Least-squares matrix: full | H atoms treated by a mixture of independent and constrained refinement |
R[F2 > 2σ(F2)] = 0.033 | w = 1/[σ2(Fo2) + (0.0155P)2 + 3.2372P] where P = (Fo2 + 2Fc2)/3 |
wR(F2) = 0.070 | (Δ/σ)max = 0.001 |
S = 1.12 | Δρmax = 0.57 e Å−3 |
2843 reflections | Δρmin = −0.40 e Å−3 |
113 parameters | Extinction correction: SHELXL2018/3 (Sheldrick 2015b) |
1 restraint | Extinction coefficient: 0.0035 (3) |
Primary atom site location: dual | |
Special details top Geometry. All esds (except the esd in the dihedral angle between two l.s. planes) are estimated using the full covariance matrix. The cell esds are taken into account individually in the estimation of esds in distances, angles and torsion angles; correlations between esds in cell parameters are only used when they are defined by crystal symmetry. An approximate (isotropic) treatment of cell esds is used for estimating esds involving l.s. planes. |
Fractional atomic coordinates and isotropic or equivalent isotropic displacement parameters (Å2) top | x | y | z | Uiso*/Ueq | Occ. (<1) |
Zn1 | 0.5 | 0.72176 (4) | 0.25 | 0.03333 (10) | |
Cl1 | 0.63307 (4) | 0.57810 (6) | 0.34859 (3) | 0.04085 (12) | |
Cl2 | 0.40494 (5) | 0.84939 (6) | 0.31868 (4) | 0.05219 (15) | |
N1 | 0.15576 (15) | 0.8071 (2) | 0.13379 (11) | 0.0396 (4) | |
H1 | 0.223 (2) | 0.770 (3) | 0.1486 (17) | 0.055 (7)* | |
C1 | 0.1482 (5) | 0.9756 (5) | 0.1328 (5) | 0.0393 (10) | 0.697 (2) |
H1A | 0.189643 | 1.011624 | 0.190345 | 0.047* | 0.697 (2) |
H1B | 0.069934 | 1.003059 | 0.118912 | 0.047* | 0.697 (2) |
C2 | 0.1923 (3) | 1.0567 (4) | 0.0703 (2) | 0.0451 (7) | 0.697 (2) |
H2A | 0.180985 | 1.163084 | 0.073337 | 0.054* | 0.697 (2) |
H2B | 0.15046 | 1.024182 | 0.012066 | 0.054* | 0.697 (2) |
Cl3 | 0.33801 (8) | 1.01959 (11) | 0.09585 (6) | 0.0569 (3) | 0.697 (2) |
C1A | 0.1726 (14) | 0.9672 (11) | 0.1224 (12) | 0.052 (4) | 0.303 (2) |
H1C | 0.200388 | 1.01761 | 0.177512 | 0.063* | 0.303 (2) |
H1D | 0.102578 | 1.01361 | 0.086803 | 0.063* | 0.303 (2) |
C2A | 0.2568 (7) | 0.9742 (9) | 0.0801 (5) | 0.0480 (18) | 0.303 (2) |
H2C | 0.229594 | 0.923437 | 0.025068 | 0.058* | 0.303 (2) |
H2D | 0.32746 | 0.929578 | 0.115896 | 0.058* | 0.303 (2) |
Cl3A | 0.2728 (3) | 1.1668 (3) | 0.06669 (18) | 0.0773 (9) | 0.303 (2) |
C3 | 0.1113 (2) | 0.7582 (3) | 0.20017 (16) | 0.0552 (6) | |
H3A | 0.034943 | 0.791657 | 0.185019 | 0.083* | |
H3B | 0.156482 | 0.799774 | 0.254694 | 0.083* | |
H3C | 0.113532 | 0.65148 | 0.203863 | 0.083* | |
C4 | 0.0928 (3) | 0.7347 (3) | 0.05022 (17) | 0.0675 (8) | |
H4A | 0.10053 | 0.628507 | 0.056467 | 0.101* | |
H4B | 0.122638 | 0.767543 | 0.007913 | 0.101* | |
H4C | 0.014696 | 0.761126 | 0.032261 | 0.101* | |
Atomic displacement parameters (Å2) top | U11 | U22 | U33 | U12 | U13 | U23 |
Zn1 | 0.02977 (15) | 0.03496 (17) | 0.03503 (17) | 0 | 0.01136 (12) | 0 |
Cl1 | 0.0366 (2) | 0.0455 (3) | 0.0358 (2) | 0.00580 (19) | 0.00738 (18) | 0.00353 (19) |
Cl2 | 0.0601 (3) | 0.0429 (3) | 0.0653 (4) | 0.0098 (2) | 0.0367 (3) | −0.0012 (2) |
N1 | 0.0331 (8) | 0.0453 (10) | 0.0401 (9) | 0.0091 (7) | 0.0129 (7) | −0.0022 (7) |
C1 | 0.042 (3) | 0.0422 (18) | 0.040 (2) | −0.0022 (15) | 0.0234 (18) | 0.0028 (14) |
C2 | 0.0476 (16) | 0.0459 (17) | 0.0437 (16) | 0.0085 (14) | 0.0186 (13) | 0.0044 (13) |
Cl3 | 0.0477 (5) | 0.0645 (6) | 0.0649 (6) | −0.0009 (4) | 0.0280 (4) | 0.0002 (4) |
C1A | 0.042 (7) | 0.083 (8) | 0.043 (6) | −0.022 (5) | 0.027 (4) | −0.003 (5) |
C2A | 0.055 (5) | 0.052 (4) | 0.042 (4) | 0.001 (4) | 0.023 (3) | 0.008 (3) |
Cl3A | 0.110 (2) | 0.0557 (13) | 0.0823 (17) | −0.0148 (13) | 0.0536 (16) | 0.0132 (12) |
C3 | 0.0694 (16) | 0.0485 (13) | 0.0532 (13) | −0.0096 (11) | 0.0288 (12) | 0.0008 (10) |
C4 | 0.0780 (19) | 0.0693 (18) | 0.0481 (13) | 0.0019 (15) | 0.0139 (13) | −0.0172 (13) |
Geometric parameters (Å, º) top Zn1—Cl2 | 2.2553 (5) | C2—H2B | 0.97 |
Zn1—Cl2i | 2.2554 (5) | C1A—C2A | 1.481 (12) |
Zn1—Cl1i | 2.2883 (5) | C1A—H1C | 0.97 |
Zn1—Cl1 | 2.2883 (5) | C1A—H1D | 0.97 |
N1—C1A | 1.476 (9) | C2A—Cl3A | 1.765 (8) |
N1—C3 | 1.480 (3) | C2A—H2C | 0.97 |
N1—C4 | 1.485 (3) | C2A—H2D | 0.97 |
N1—C1 | 1.515 (5) | C3—H3A | 0.96 |
N1—H1 | 0.87 (3) | C3—H3B | 0.96 |
C1—C2 | 1.535 (5) | C3—H3C | 0.96 |
C1—H1A | 0.97 | C4—H4A | 0.96 |
C1—H1B | 0.97 | C4—H4B | 0.96 |
C2—Cl3 | 1.779 (3) | C4—H4C | 0.96 |
C2—H2A | 0.97 | | |
| | | |
Cl2—Zn1—Cl2i | 118.93 (3) | H2A—C2—H2B | 108.1 |
Cl2—Zn1—Cl1i | 104.94 (2) | N1—C1A—C2A | 105.4 (7) |
Cl2i—Zn1—Cl1i | 108.36 (2) | N1—C1A—H1C | 110.7 |
Cl2—Zn1—Cl1 | 108.36 (2) | C2A—C1A—H1C | 110.7 |
Cl2i—Zn1—Cl1 | 104.94 (2) | N1—C1A—H1D | 110.7 |
Cl1i—Zn1—Cl1 | 111.38 (3) | C2A—C1A—H1D | 110.7 |
C1A—N1—C3 | 119.8 (5) | H1C—C1A—H1D | 108.8 |
C1A—N1—C4 | 111.1 (8) | C1A—C2A—Cl3A | 103.8 (6) |
C3—N1—C4 | 110.5 (2) | C1A—C2A—H2C | 111 |
C3—N1—C1 | 105.3 (2) | Cl3A—C2A—H2C | 111 |
C4—N1—C1 | 114.6 (3) | C1A—C2A—H2D | 111 |
C1A—N1—H1 | 103.7 (19) | Cl3A—C2A—H2D | 111 |
C3—N1—H1 | 106.0 (18) | H2C—C2A—H2D | 109 |
C4—N1—H1 | 104.1 (18) | N1—C3—H3A | 109.5 |
C1—N1—H1 | 116.1 (18) | N1—C3—H3B | 109.5 |
N1—C1—C2 | 116.3 (4) | H3A—C3—H3B | 109.5 |
N1—C1—H1A | 108.2 | N1—C3—H3C | 109.5 |
C2—C1—H1A | 108.2 | H3A—C3—H3C | 109.5 |
N1—C1—H1B | 108.2 | H3B—C3—H3C | 109.5 |
C2—C1—H1B | 108.2 | N1—C4—H4A | 109.5 |
H1A—C1—H1B | 107.4 | N1—C4—H4B | 109.5 |
C1—C2—Cl3 | 110.7 (3) | H4A—C4—H4B | 109.5 |
C1—C2—H2A | 109.5 | N1—C4—H4C | 109.5 |
Cl3—C2—H2A | 109.5 | H4A—C4—H4C | 109.5 |
C1—C2—H2B | 109.5 | H4B—C4—H4C | 109.5 |
Cl3—C2—H2B | 109.5 | | |
| | | |
C3—N1—C1—C2 | 178.5 (4) | C3—N1—C1A—C2A | 157.7 (7) |
C4—N1—C1—C2 | −59.9 (6) | C4—N1—C1A—C2A | −71.4 (12) |
N1—C1—C2—Cl3 | −61.3 (6) | N1—C1A—C2A—Cl3A | 179.3 (9) |
Symmetry code: (i) −x+1, y, −z+1/2. |
Hydrogen-bond geometry (Å, º) top D—H···A | D—H | H···A | D···A | D—H···A |
N1—H1···Cl1i | 0.87 (3) | 2.51 (3) | 3.3124 (18) | 156 (2) |
N1—H1···Cl2 | 0.87 (3) | 3.03 (3) | 3.5620 (19) | 121 (2) |
Symmetry code: (i) −x+1, y, −z+1/2. |
Acknowledgements
The authors thank Ms Annette Drury for assistance in preparation of the ZnCl42− salt.
References
Andrews, P. C., Gardiner, M. G., Raston, C. L. & Tolhurst, V.-A. (1997). Inorg. Chim. Acta, 259, 249–255. CSD CrossRef CAS Web of Science Google Scholar
Bond, M. R. & Silwal, S. (2023). IUCrData, 8, x230015. Google Scholar
Bruker (2017). APEX3 and SAINT. Bruker AXS Inc., Madison, Wisconsin, USA. Google Scholar
Farrugia, L. J. (2012). J. Appl. Cryst. 45, 849–854. Web of Science CrossRef CAS IUCr Journals Google Scholar
Groom, C. R., Bruno, I. J., Lightfoot, M. P. & Ward, S. C. (2016). Acta Cryst. B72, 171–179. Web of Science CrossRef IUCr Journals Google Scholar
Krause, L., Herbst-Irmer, R., Sheldrick, G. M. & Stalke, D. (2015). J. Appl. Cryst. 48, 3–10. Web of Science CSD CrossRef ICSD CAS IUCr Journals Google Scholar
Marchetti, F., Pampaloni, G. & Zacchini, S. (2015). Polyhedron, 85, 369–375. Web of Science CSD CrossRef CAS Google Scholar
Muller, K., Hosten, E. C. & Betz, R. (2021). Z. Kristallogr. New Cryst. Struct. 236, 287–289. Web of Science CSD CrossRef CAS Google Scholar
Rodrigues Silva, D., de Azevedo Santos, L., Hamlin, T. A., Fonseca Guerra, C., Freitas, M. P. & Bickelhaupt, F. M. (2021). ChemPhysChem, 22, 641–648. Web of Science CrossRef CAS PubMed Google Scholar
Schmidt, M. W., Baldridge, K. K., Boatz, J. A., Elbert, S. T., Gordon, M. S., Jensen, J. H., Koseki, S., Matsunaga, N., Nguyen, K. A., Su, S., Windus, T. L., Dupuis, M. & Montgomery, J. A. (1993). J. Comput. Chem. 14, 1347–1363. CrossRef CAS Web of Science Google Scholar
Sheldrick, G. M. (2015a). Acta Cryst. A71, 3–8. Web of Science CrossRef IUCr Journals Google Scholar
Sheldrick, G. M. (2015b). Acta Cryst. C71, 3–8. Web of Science CrossRef IUCr Journals Google Scholar
Westrip, S. P. (2010). J. Appl. Cryst. 43, 920–925. Web of Science CrossRef CAS IUCr Journals Google Scholar
Wolfe, S. (1972). Acc. Chem. Res. 5, 102–111. CrossRef CAS Web of Science Google Scholar
This is an open-access article distributed under the terms of the Creative Commons Attribution (CC-BY) Licence, which permits unrestricted use, distribution, and reproduction in any medium, provided the original authors and source are cited.
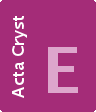 | CRYSTALLOGRAPHIC COMMUNICATIONS |
ISSN: 2056-9890
Open

access